The Carbon Footprint of Aluminum Fenestration
A Study of Key Factors Contributing to Embodied and Operational Carbon
Presented on October 12, 2022 at Facade Tectonics 2022 World Congress
Sign in and Register
Create an Account
Overview
Abstract
The historical focus on reducing the carbon footprint of a building has recently shifted to include more emphasis on embodied carbon, the carbon emitted in the construction of the building, the upstream manufacturing, and raw materials extraction. Materials comprising the building envelope have significant contributions from embodied and operational carbon, thus architects and engineers must consider both aspects to make optimal decisions for minimizing carbon dioxide emissions. Here we perform a parametric study of the total carbon footprint of an aluminum fenestration system and the resulting percentage of embodied carbon. We vary five parameters: the aluminum recycled content, the primary aluminum ingot source, the thermal performance of the fenestration system, the climate zone of the building, and the carbon intensity of the grid at the building to calculate 1,296 permutations of carbon dioxide emissions. We perform energy modeling simulations on an ASHRAE medium office model building in Buffalo, NY and Tucson, AZ and employ an attribution method to calculate the energy consumed due to heat loss/gain through the fenestration system. We find that the embodied carbon content of the fenestration system can vary dramatically from 13 to 77% of the total carbon with a median value of 34%. Operational carbon can vary by a factor of 13 while embodied carbon can vary by a factor of three. These results provide a strong indication that carbon reduction strategies related to the building envelope need to adopt a holistic approach, calculating both embodied and operational carbon for any specific building.
Authors
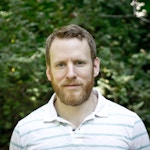
Tom Bougher
Director of Applied Research
Oldcastle BuildingEnvelope
tbougher@obe.com
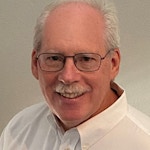
Richard Braunstein
Vice President of R&D
Oldcastle BuildingEnvelope
RBraunstein@obe.com
Keywords
1. Introduction
Issues surrounding climate change through the accumulation of additional carbon in our natural environment may very well be the challenge of this generation. Considering the built environment consumes approximately two-thirds of all electricity, any stakeholder within this sector, including the architect, building material supplier, and their downstream supply chains, has an inherent responsibility to find ways to reduce this demand. All sources of carbon generation are contributing to this problem, but there has been a recent shift that focuses on embodied carbon in particular. One study estimates that embodied carbon accounts for approximately 50% of total carbon for newly constructed buildings from 2020 to 2040 (Architecture2030, 2021). Another study of over 5,000 theoretical buildings showed that the majority had less than 15% embodied carbon, while a small subset of buildings had embodied carbon of 50-80% (Basbagill, 2013). Clearly, an architect’s design and material choices will be dramatically different for a scenario with 15% embodied carbon versus 80%. It is crucial that we can accurately estimate operational versus embodied carbon to help architects and engineers make optimum choices with respect to environmental impact. This evolution in thinking is a departure from the prior wisdom which generally accepted that the majority of carbon generation came from the building operation. Based on this earlier assessment, carbon reduction strategies focused on the development and specification of high-performance, thermally efficient glass and framing fenestration products designed to lower a building’s operational carbon. However, for higher levels of embodied carbon, architects must take a deeper dive into the sourcing of a building’s materials. After all, this is a fixed quantity once the construction of the building is complete as opposed to operational carbon which has the potential of reduction with the greening of the power grid. This shift in thinking could have major consequences on the building design, but not all of which will lead to a reduction in carbon.
Here we investigate the relative contributions of embodied and operational carbon to a commercial building specifically from the fenestration system. We choose five key parameters to vary within practical ranges and calculate the resulting embodied carbon content with 1,296 permutations. The results can lead to a better understanding of how best to reduce the total carbon footprint of a fenestration system under different scenarios.
2. Methodology
In this work, we aim to assess the contributions of operational and embodied carbon for an aluminum and glass fenestration system and how that is affected by various factors during
Access Restricted
3. Results and Discussion
To assess the model building operation in each climate zone, we simulate each building using the ASHRAE 2019 fenestration system in conjunction with the electrical grid from each state. The
Access Restricted
4. Conclusions
In this work, we performed an analysis of a fenestration system and commercial building and attributed HVAC energy consumption to the fenestration system in such a way to compare the
Access Restricted
Acknowledgements
The authors would like to thank Helen Sanders for her helpful discussions on this work.
Rights and Permissions
Aluminum Extruders Council Aluminum Extrusions (2016). Environmental Product Declaration. Declaration Number 11240237.101.1.
Architecture 2030, https://architecture2030.org/why-the-building-sector/, accessed October 22, 2021.
Basbagill, J.P. (2013) Integration of life cycle assessment and conceptual building design, PhD dissertation, Stanford University.
Lewis, M., Huang, M., Carlisle, S., Simonen, K. Part II: Measuring embodied carbon. AIA-CLF Embodied Carbon Toolkit for Architects.
Milovanoff, A., Posen, I. D., & MacLean, H. L. (2021). Quantifying environmental impacts of primary aluminum ingot production and consumption: A trade‐linked multilevel life cycle assessment. Journal of Industrial Ecology, 25(1), 67-78.
Prototype Model Buildings (2021). Building Energy Codes Program, https://www.energycodes.gov/prototype-building-models accessed October 4, 2021.
Saadatian, S.; Freire, F.; and Simoes, N. 2021, “Embodied impacts of window systems: A comparative assessment of framing and glazing alternatives,” Journal of Building Engineering, 35, 102042, DOI: 10.1016/j.jobe.2020.102042
Sinha, A., and Kutnar, A. 2012. “Carbon Footprint versus Performance of Aluminum, Plastic, and Wood Window Frames from Cradle to Gate,” buildings, 2, pp. 542-553, DOI: 10.3390/buildings2040542
US Energy Information Administration. (2021) How much carbon dioxide is produced per kilowatthour of U.S. electricity generation?, https://www.eia.gov/tools/faqs/faq.php?id=74&t=11 accessed December 27, 2021.
US Energy Information Administration. (2021) Annual Energy Outlook 2021, https://www.eia.gov/outlooks/aeo/ release date February 3, 2021.
US Energy Information Administration. (2021) State Electricity Profiles 2020, https://www.eia.gov/electricity/state/newyork/ accessed November 6, 2021.
Vitro Architectural Glass Processed Glass Products (2017). Environmental Product Declaration. ASTM-EPD #062. https://www.vitroglazings.com/media/x4ajs3s2/vitro-epd-processed-glass-products.pdf