Spandrel Thermal Simulation Techniques
Identifying shortfalls of procedures to determine an applicable tool
Presented on October 10, 2024 at Facade Tectonics 2024 World Congress
Sign in and Register
Create an Account
Overview
Abstract
Glazed wall systems, such as curtain walls and window walls, are one of the most commonly used façade systems in modern buildings in North America. These systems consist of transparent and opaque areas, which allow natural light, views, and solar gains through the transparent sections, as well as thermal resistance and concealing interior building components covered by the opaque sections. While the thermal and optical performance of the transparent areas is very well understood and evaluated through industry standards such as NFRC-100 and 200, the thermal performance of the opaque sections of glazed wall systems, also known as spandrel assemblies, is not.
The process of calculating heat loss through spandrel assemblies is complicated due to thermal bridging caused by highly conductive mullions and framing members. This makes it challenging to evaluate using traditional 1D or 2D calculations. While thermal bridging is also present in transparent sections, the additional heat loss near the center of the spandrel assembly is different. Using similar evaluation techniques can lead to misleading results. Studies reveal significant differences in the performance of current simulation methods for spandrel assemblies, which can diverge up to 20% compared to laboratory testing. This divergence has caused many designers to believe their systems perform better than they do, resulting in increased heating and cooling demand and condensation problems. This uncertainty also poses a risk for compliance with more stringent energy code requirements. As building codes in North America are reducing energy demand requirements to lower operational greenhouse gas emissions through lower facade U-factors, there is a need for more accurate methods to evaluate facade systems to reflect their actual thermal performance.
This paper compares various thermal simulation techniques for evaluating the thermal performance of spandrel assemblies in typical curtain wall systems with variations for glass types, insulation levels, and cladding, among others. These include 2D and 3D simulation methods:
- NFRC-100 standard for fenestration that is commonly adopted by the industry.
- Recently published NFRC 100 new guidelines for spandrel assemblies
- FEN-BC/FGIA Reference guideline
- 3D thermal simulations following CSA Z5010
This paper aims to highlight the differences in thermal simulation results for spandrel assemblies in terms of overall heat loss (U-factors) and surface temperatures to evaluate condensation risks. Results aid in identifying shortfalls of procedures to determine the right tools for the correct application.
Authors
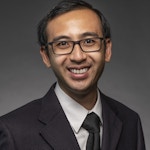
Ivan Lee
Building Science Consultant
Morrison Hershfield now Stantec
ilee@morrisonhershfield.com
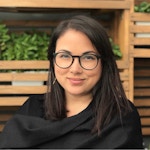
Edlyn Garcia La Torre
Facade Consulant
Morrison Hershfield now Stantec
egarcialatorre@morrisonhershfield.com
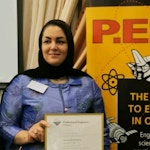
Shahima Rahmatipour
Building Science Consultant
Morrison Hershfield now Stantec
srahmatipour@morrisonhershfield.com
Keywords
Paper content
Objective: Better evaluate thermal performance of spandrels in buildings to help achieve more accurate building energy and carbon performance.
Problem Statement: Many spandrel assemblies are not accurately evaluated which
Access Restricted
Rights and Permissions
American Society of Heating, Refrigerating and Air Conditioning Engineers Inc., ASHRAE “Research Project Report RP-1365 Thermal Performance of Buildings Envelope Details for Mid and High-Rise Buildings”, 2011
BC Hydro, “Building Envelope Thermal Bridging Guide version 1.6”, BC Hydro, 2021
Bettenhausen, D. W., L.D. Carbary, C.K. Boswell, O.C. Brouard, J.R. Casper, S. Yee and M.M. Fukutome, “A Comparison of Thermal Transmittance of Curtain Wall Spandrel Areas Employing Mineral Wool and Vaccum Insulation Panels by Numerical Modeling and Experimental Evaluation”, Glass Performanec Days, Tampre, Finland, 2015
Boafo, F.E., J.H. Kim, and J.T. Kim, “Numerical Study of Slim Curtain Wall Spandrel with Integrated Vaccuum Insulated Panel: Concept, Performance Evaluation and Challenges”, Energy and Buildings, Vol. 183, 2018, 139-150.
Charles Pankow Foundation, “Thermal Performance of Spandrel Assemblies in Glazed Wall Systems”, Charles Pankow Foundation Research Grant RGA #04-22, 2023
CSA Group, “CSA Z5010: Thermal Bridging Calculation Methodology”, CSA Group, 2021
Dunlap, A., R. Asava, and K. Gross, “Three Dimensional Effects on the Thermal Performance of Insulated Curtain Wall Spandrels”, Building Enclosure Science & Technology Conference, Philadelphia, PA, 2020
Fenestration Association of British Columbia, “Reference Procedure for Simulating Spandrel U-Factors", Fenestration Association of British Columbia, Surrey, British Columbia, 2017
Fenestration Association of British Columbia, “User Guide to Reference Procedure for Simulating Spandrel U-Factors", Fenestration Association of British Columbia, Surrey, British Columbia, 2017
Jackson, J.A., C.M. Saldanha, G. Guldentops, J.R. Yap, R.J. Abdallah, and S.B. Rentfro, “Thermal Performance of Spandrel Assemblies in Glazing Systems”, in Building Science and the Physics of Building Enclosure Performance, D. Lemieux and J. Keegan, eds., ASTM International, West Conshohocken, PA, 2020: 450-480
National Fenestration Rating Council, “ANSI/NFRC 100- 2023 Procedure for Determining Fenestration Product U-factors",2023
National Fenestration Rating Council, “Therm 7/ Window 7 NFRC Simulation Manual”, 2023
Norris, N., L. Carbary, S. Yee, P. Roppel, and P. Ciantar, “The Reality of Quantifying Curtain Wall Spandrel Thermal Performance: 2D, 3D, and Hotbox Testing”, Building Enclosure Science & Technology (BEST4) Conference, Kanas City, MO, 2015