Mapping Advanced Facades
Creating a Building Taxonomy and Documenting Global Case Studies
Presented on October 9, 2024 at Facade Tectonics 2024 World Congress
Sign in and Register
Create an Account
Overview
Abstract
This paper describes the development of an interactive map that highlights case studies of advanced facade design strategies and technologies from around the world. The overarching goal of this project is to disseminate information about best practices for climate responsive building design as showcased through facades. The non-profit project, led by the Center for the Built Environment at UC Berkeley and the Polytechnic University of Bari, Italy, was launched in 2020 and aims to serve design professionals, students, academics and others.
This curation process required the authors to grapple with numerous subjective and objective measures, and defining exactly what constitutes a high-performing facade. (Can a glass curtainwall in a desert climate ever be considered an appropriate solution?) The authors deliberated about what performance metrics can be applied in the selection process, while also allowing for a wide range of innovative and diverse examples. The authors ultimately focused on nine facade considerations relevant to the creation of sustainable and comfortable buildings: daylight and solar control, natural ventilation, noise control, embodied carbon, energy generation, and innovative insulation systems.
An additional goal of this project has been to include as much detailed technical information as possible. As part of this process, the authors devised a comprehensive taxonomy of building and facade data. This taxonomy has been integrated into the map and website design, so users can filter the case study projects based on the characteristics of interest or relevance to a specific investigation or design question. To capture such detail, an online form allows design team members, who are generally the gatekeepers of such technical details, to directly and conveniently provide project information. Such information has been provided by team members from firms such as Foster + Partners, DIALOG and Transsolar/KlimaEngineering.
The project has been online since 2021, and the project team continues to identify and add projects, at https://facademap.cbe.berkeley.edu/.
Authors
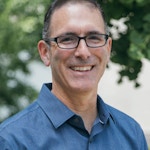
David Russell Lehrer
CBE's Communications Director
University of California, Berkeley
lehrer@berkeley.edu
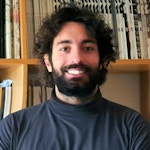
Vito Lamberti
Visiting Ph.D. Student
University of California, Berkeley
v.lamberti@phd.poliba.it
Keywords
Paper content
Introduction and Background
To make progress towards mitigation of climate change and managing local environmental impacts, it’s clear that broad and significant technological and policy advances are required for the
Access Restricted
Acknowledgements
The authors wish to thank Giovanni Betti for his contributions towards the development of the facade taxonomy, identifying case studies and providing details about the Bloomberg European Headquarters. We also thank Minghao Xu and Yunzhu Ji for documenting projects, Paul Raftery for his initial conception of a facade map, and Tom Parkinson for his early participation. We also thank the many Technical Advisory Group members, and design team members for contributing design specifications and data.
Rights and Permissions
Aksamija, A., Perkins+Will, (2013). Sustainable Facades: Design Methods for High-Performance Building Envelopes, John Wiley & Sons, Inc., Hoboken, New Jersey, ISBN: 978-1-118-45860-0
Karmann, C., Schiavon, S., & Bauman, F. (2014). Online Map of Buildings Using Radiant Technologies. UC Berkeley: Center for the Built Environment. Retrieved from https://escholarship.org/uc/item/9rs8t4wb
Michael, M., Favoino, F., Jin, Q., Luna-Navarro, A., & Overend, M. A Systematic Review and Classification of Glazing Technologies for Building Facades. Energies 2023, 16, 5357. https://doi.org/10.3390/en16145357
Ko, W., Michael, G. Kent, Schiavon, S., Levitt, B. & Betti, G. (2022). A Window View Quality Assessment Framework, LEUKOS, 18:3, 268-293, DOI: 10.1080/15502724.2021.1965889
Ko, W., Schiavon, S., Brager, G., & Levitt, B. (2018). Ventilation, Thermal and Luminous Autonomy Metrics for am Integrated Design Process. UC Berkeley: Center for the Built Environment. http://dx.doi.org/10.1016/j.buildenv.2018.08.038
Romano, R., Aelenei, L., Aelenei, D., & Mazzucchelli, E. S. (2018). What is an Adaptive Facade? Analysis of Recent Terms and Definitions from an International Perspective. Journal of Facade Design and Engineering, 6(3), 65–76.https://doi.org/10.7480/jfde.2018.3.2478
Shan, R., & Junghans, L., (2023). Multi-Objective Optimization for High-Performance Building Facade Design: A Systematic Literature Review. Sustainability. 2023; 15(21):15596. https://doi.org/10.3390/su152115596
Zelenay, K., Perepelitza, M., & Lehrer, D. (2011). High-Performance Facades Design Strategies and Applications in North America and Northern Europe. UC Berkeley: Center for the Built Environment. Retrieved from https://escholarship.org/uc/item/4vq936rc