Investigating Heat Development in Shadow Box Façade Systems
A Mock-up Test Approach for Follow-up Study
Presented on October 10, 2024 at Facade Tectonics 2024 World Congress
Sign in and Register
Create an Account
Overview
Abstract
The prevalence of fully glazed facades in modern office buildings has been steadily increasing, primarily driven by architects' focus on aesthetic appeal. While the desire for fully glazed designs with seamless transitions across buildings is evident, the shadow box, which consists of two layers of glass and an opaque back pan with an air cavity in between, stands out among other spandrel panel applications due to its unique visual effect on the facade. Architects frequently opt for the shadow box in designing the opaque sections of modern glass facades because these applications provide them with a high degree of design freedom. However, the shadow box is a complex system that requires precise design and manufacturing to prevent any negative impact on the overall building performance. It is susceptible to issues such as condensation, contamination, and overheating, which give rise to project-specific challenges for engineers and designers, adding complexity to detailing and real-life applications. This research constitutes the second phase of the study previously published for the ISCCGF – Zagreb 2023 Conference. Building upon the earlier work, this follow-up study employs a mock-up test approach to investigate the issue of overheating in shadow box systems.
The primary aim is to conduct extensive analysis of the underlying reasons for this problem. This will be achieved by utilizing the same test units while expanding both the dataset and the observation period. While the initial study mainly focused on type 3 shadow box behaviour, this current study addresses all shadow box types, drawing comparisons between them and re-examining the type 3 findings within a longer timeframe. The findings are expected to have considerable impact on future shadow box applications. Thus, the overall goal is to examine the behaviour of different shadow box settings and provide valuable information to the industry to overcome the potential problems faced in most projects. Moreover, the long-term goal is to contribute to the development of an enforceable consensus on shadow box applications in the industry, which currently does not exist.
Authors
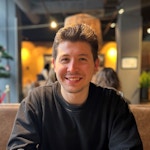
Ahmet Faruk Cakir
Master's Student in Facade Engineering and Werkstudent at Schüco
TH OWL
ahmet.cakir@stud.th-owl.de
Prof. Daniel Arztmann
Head of Custom Design International and Professor at TH OWL
Schüco International KG
darztmann@schueco.com
Keywords
Paper content
INTRODUCTION AND BACKGROUND
Curtain wall is a prominent component of contemporary architectural practice, as it gives an opportunity for architects to blend aesthetics and high-performance (Murray 2009). These systems comprise sections that can be transparent, translucent, or opaque (Charles Pankow Foundation 2023). Designers often opt for a shadow box design when incorporating glass into spandrel sections to achieve visual continuity between opaque and transparent zones (Kragh 2014). The shadow box design typically involves using the same type of glass found in the vision areas and features an architecturally finished panel behind the glass, providing an expression of visual depth in comparison to back-painted spandrel glass or glazed-in metal panels (Walsch 2018).
The concept of the "shadow box" finds its roots in the use of shallow glass cases for displaying memorabilia, such as medals and diplomas (Brzezicki 2014). Initially designed to aesthetically present tangible achievements and personal accomplishments, the shadow box has then evolved over time along with the technological advancements. Cucuzzella, Rahimi, and Soulikias (2022) described that façade design and construction were a site of intensive experimentation and innovation in façade design throughout the 20th century, drove architects and engineers to develop construction techniques such as shadow box in the 1960s and 1970s (Brzezicki 2014).
Shadow boxes are the non-vision area of the curtain wall and are typically 1500mm (a module width) by 600-1500mm tall which depends on the designer’s desired window to wall ratio for the building (CTBUH 2014). The assembly consists of the glass unit and the opaque back pan to create a perception of depth with an air cavity layer in between, resembling the design of a shallow glass case. The air cavity between the glass and opaque back panel ranges from 50 mm to 100 mm (CWCT 2014), depending on aesthetic and energy performance requirements.
Figure 1. The basic components of the shadow box. (Arztmann 2016).
The frame of facade profiles is constructed from aluminum or steel, and the glass used for shadow boxes ranges from single to triple-glazed with tinted or clear panes, based on project-specific requirements (CWCT 2014). The opaque back panel is thermally insulated and covered by a vapor barrier to isolate the shadow box assembly and insulation from the internal environment (McCowan 2008). The opaque back panel offers a spectrum of materials, such as anodized or painted aluminum, steel sheet, ceramic coated glass, fabric, or even wood (Boswell 2005).
Despite the architectural significance and benefits of shadow box constructions, the shadow box system is complex, requiring precise design and manufacturing to ensure it doesn't negatively impact the overall performance of the façade. The system is susceptible to challenges outlined in the literature, such as condensation, overheating, thermal bridging, and contamination, which pose specific challenges for engineers and designers, complicating real-world applications as well as decreasing reliability to the system (Lang 2010; Bauer, Bäumler, and Bauer 2015).
Arztmann (2016) highlighted the challenge of overheating in shadow box applications, where solar energy passing through the glass layer contacts the opaque back panel, elevating the air cavity temperature. The cavity air can reach 80°C or higher (CWCT 2014), and Singh, Arztmann, and Balderrama (2023) reported temperatures as high as 104°C in the initial phase of the study. Such elevated temperatures, especially in the absence of thermal separation from adjacent assemblies, can affect the surface temperatures on the surrounding profiles and inner vapor barrier, specifically if they are made from sheet metal or other thermal conducting materials (Arztmann 2016). Contamination and staining within shadow boxes become critical issue, especially when considering overheating. Elevated temperatures can lead to substantial contamination from materials within the shadow box, potentially releasing off-gases and affecting the overall appearance of the overall aesthetic integrity of the façade (Lang, 2010).
Figure 2. Summary of issues known from shadow box projects. (Arztmann 2016).
The objective is to examine different shadow box settings, offering insights into project challenges and re-evaluating findings of initial study to enhance understanding of persistent issues in the literature. This research, therefore, extends a prior study presented at the ISCCGF – Zagreb 2023 Conference, which focused on overheating issues in shadow box facade systems. To achieve this, we adopted a mock-up test approach, utilizing the same test units as the initial study but expanding both the dataset and observation period. The cumulative findings from this follow-up research and the initial study will form the basis for developing effective solutions to address overheating issues in shadow box systems. Ultimately, our aim is to contribute to the establishment of an enforceable consensus on the optimal applications of shadow boxes within the industry, which currently lacks a standardized framework.
METHODOLOGY
In this chapter, we outline the methodology employed to investigate the thermal behaviour of shadow box façade systems. The research approach relies on a comprehensive experimental setup using mock-up units. To ensure the reliability and accuracy of the study, various factors, including location selection, sensor integration, and the chosen shadow box assemblies, are detailed.
EXPERIMENTAL SETUP
LOCATION SELECTION
The experimental setup took place on the rooftop of the car park situated at the Schüco Campus in Bielefeld, Germany. This location was chosen for its strategic positioning, ensuring that the test unit would be exposed to direct sunlight without any obstructions. To maximize solar exposure, the test unit was oriented to face south, thereby optimizing its reception of solar radiation. Moreover, A dedicated test chamber was incorporated behind the shadow boxes to regulate and maintain the required interior environment, ensuring consistency in testing conditions.
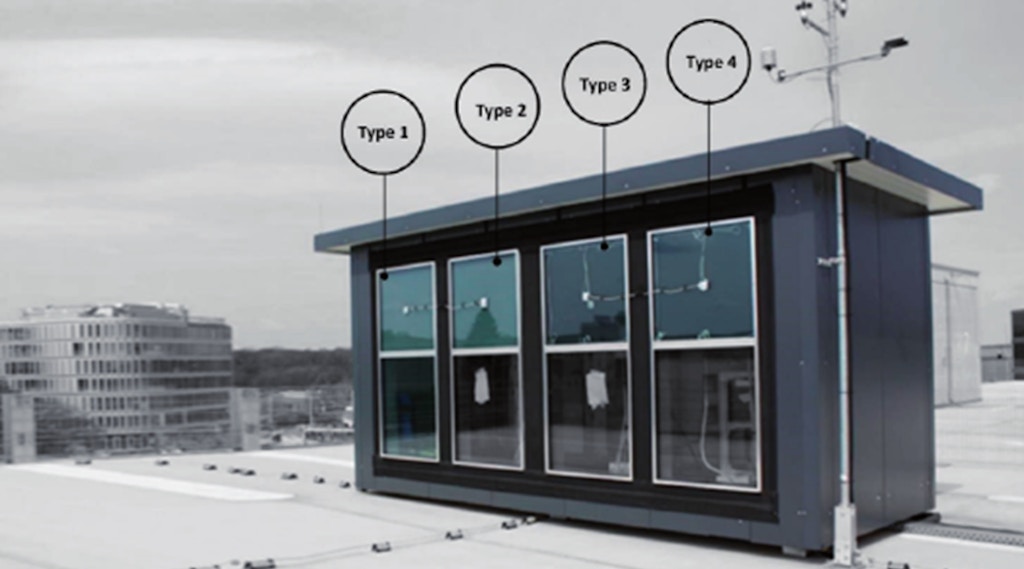
Figure 3. Mock-up setup, Schüco Campus, Bielefeld.
SENSOR INTEGRATION
To capture comprehensive weather data and enable a thorough analysis of the thermal performance, a set of sensors was integrated into the test unit. To measure the instant weather data, the sensors included the following components:
Pyranometer: This device was employed to measure global irradiation levels, providing data on solar energy input.
Wind Speed Sensor: This sensor recorded wind speed data, contributing to the evaluation of external factors affecting the shadow box units.
Rainfall Sensor: A sensor for measuring raindrops was utilized to monitor precipitation levels, an essential factor in understanding the impact of weather conditions on thermal performance.
To measure the thermal performance of shadow box, the shadow box related sensors included the following components:
Surface Temperature of Glass (TA): A sensor on the outermost surface of the glass assembly. There is only one on the centre of the outermost glass pane.
Surface Temperature of Glass (TI): A sensor on the innermost surface of the glass assembly. There are 5 of them; 2 on the top, 1 on the centre, 2 on the bottom.
Air Temperature Sensor (TL): A sensor situated inside the cavity to record the air temperature of the cavity. There are 5 of them; 2 on the top, 1 on the centre, 2 on the bottom.
Surface Temperature of Panel (PTI): A sensor on the back pan metal sheet to measure surface temperature. There are 5 of them; 2 on the top, 1 on the centre, 2 on the bottom.
Surface Temperature of Panel / Profile (RT): A sensor on inner surfaces of aluminium profiles to measure the surface temperature.There are 3 of them; 2 on the aluminium profiles 1 on the innermost aluminum panel.
Relative Humidity (HL): A sensor to measure the relative humidity in the air.
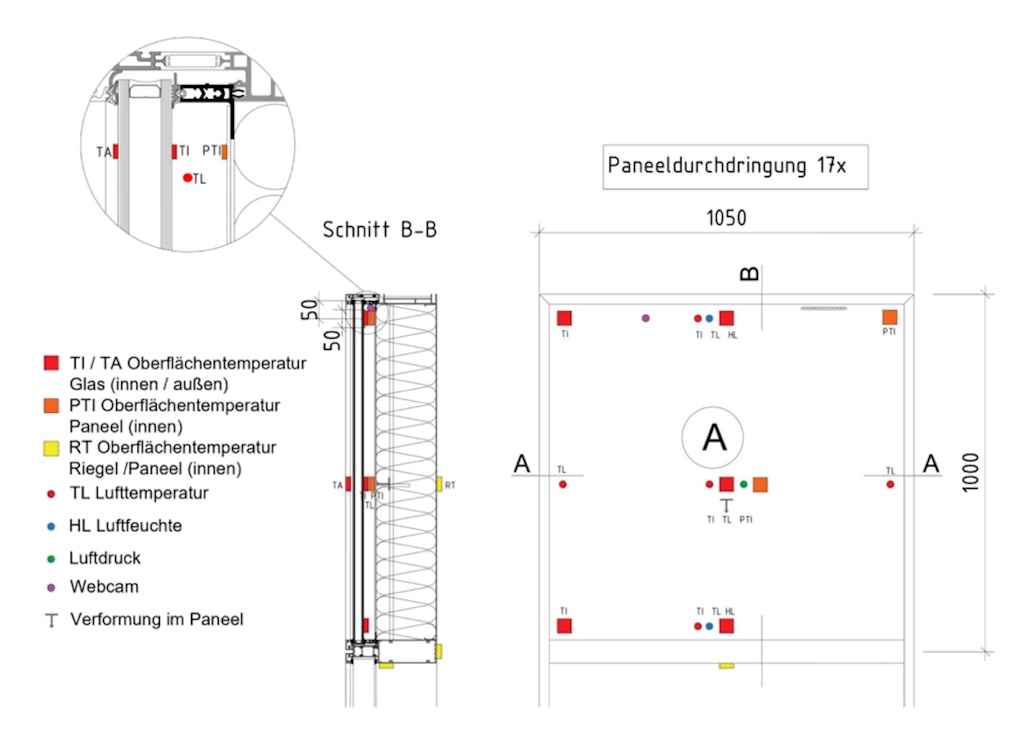
Figure 4. Type 3 Shadow Box’s Mock-up setup, Demonstration of Sensors.
SHADOW BOX TEST UNIT TYPES
Recognizing the diversity of shadow box applications in the industry, our research considered the varying geographical influences, design limitations, and local norms and regulations. To provide a comprehensive analysis, four of the most used shadow box assemblies were selected for examination. The thermal performance of these assemblies was assessed over a duration spanning from May 2021 to March 2023, facilitating an in-depth evaluation of their efficiency and differences.
The selected shadow box assemblies include; Pressure Equalized without an Air Cavity, Vented with an Opacifier on the Fourth Surface of DGI (Double Glazing Insulated), Vented with Transparent DGI, Pressure Equalized with Transparent DGI.
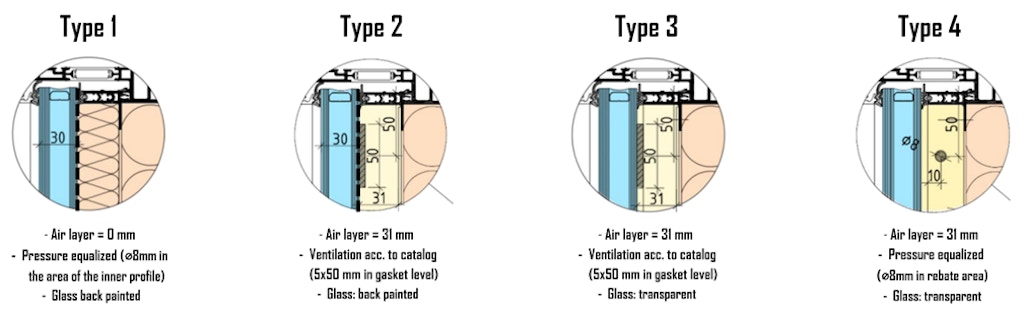
Figure 5. Shadow box types used for mock-up test.
DATA PROCESSING METHODOLOGY
The methodology for data analysis and processing is a crucial component of this research, aiming to extract accurate insights from the extensive datasets generated by the sensors over a nearly three-year test period. This sub-chapter outlines the steps involved in collecting, organizing, and refining the data to ensure that the results are robust and reliable. The initial stage of data analysis involves the collection of data from the sensors detailed in the previous section. The recorded data points encompass a wide range of weather-related parameters, including global irradiation, wind speed, rainfall, and interior conditions. These data points are recorded on a monthly basis to cover the entire duration of the study, which spans almost three years.
As part of the data processing procedure, the monthly datasets are consolidated through the use of Excel Power Query. This consolidation is essential given the extended duration of the test period. During this process, various data anomalies, such as standard deviations and occasional sensor malfunctions, are addressed. The datasets are meticulously organized, or "manipulated," to mitigate the impact of these anomalies and ensure that the results accurately reflect the conditions under investigation. To eliminate deviation values and address data inconsistencies, additional code lines are integrated into the query. This crucial step significantly contributes to the production of reliable and consistent datasets that are integral to the subsequent analysis. In addition to provide accuracy, this contribution enable us to filter out data for picking the dataset required for each data interpretation.
Figure 6. Excel Power Query Advanced Editor.
RESULTS
This chapter presents the results of the mock-up test approach conducted to investigate heat development in shadow box façade systems. The research aims to analyse and understand the underlying reasons for overheating in shadow box systems by expanding the dataset and observation period beyond the initial study conducted for the ISCCGF – Zagreb 2023 Conference. The results are structured to address the behaviour of different shadow box types, compare them, and re-examine the findings related to type 3 shadow boxes within a longer timeframe.
TEMPERATURE FLUCTUATION TREND FOR ALL TYPES
The graph below shows how the cavity temperatures changed in four different shadow box test models during the entire testing period. The black curve represents the trendline, which closely follows the directional changes in temperature, illustrating its fluctuation. This line helps to understand the overall direction in which temperatures are moving over time. To get an accurate picture, it's essential to create this line without including values that deviate significantly from the average since including these unusual values could lead to misinterpreting the heat build-up.
For precise data analysis, the data chart is divided into segments in time as figure 8 shows, taking into account significant events such as equinoxes (spring and autumn) and solstices (summer and winter) during the testing period. The equinoxes and solstices are of importance in terms of solar incident angle since they define the range of sun angles, including maximum, minimum, and median angles during a year. This segmentation helps identify the key turning points of the trendline and enables the comparison of temperature fluctuations within the same time period across consecutive “segments” and years.
In this analysis, we focus on the TI sensor, specifically TI_3, which is located in the middle of the glass pane within each test unit. As figure 7 shows, Type 3 shadow boxes exhibit the highest temperatures during the testing period, followed by Type 2, Type 4, and Type 1. It's important to note that there is no consistent superiority among shadow boxes, especially for types other than Type 3, as their temperature trends may sometimes overlap, alternate, or surpass each other.
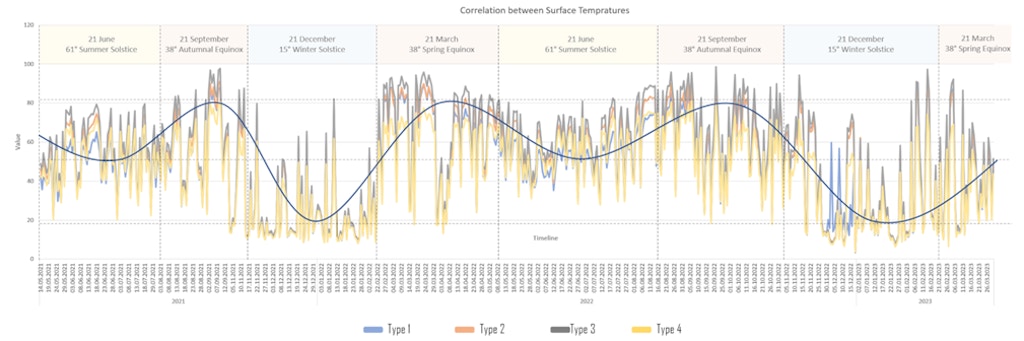
Figure 7. Temperature Fluctuation Trend for All Types.
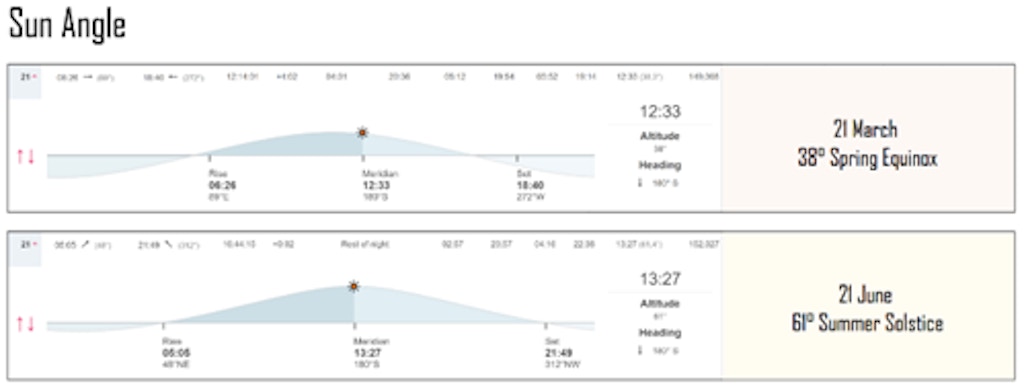
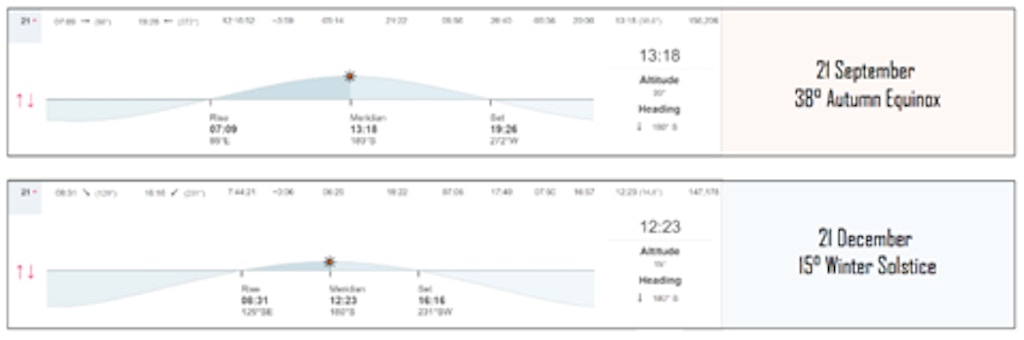
Figure 8. Sun Angles according to different Periods, (“Sunrise and Sunset Times in Berlin, June 2022,” n.d.).
THE ROLE OF SUN INCIDENT ANGLE
The previous graph provided a broad overview of the temperature patterns across all shadow box configurations during the study period. However, it lacked a detailed examination of the influence of sun angle. Consequently, the subsequent graph was created to address this gap, specifically delving into the effects of sun angle. The focus of this analysis was on the Type 3 shadow box test unit, known for consistently demonstrating the poorest temperature performance throughout the testing period. The analysis focused on comparing temperature differences at the top and centre positions between PTI sensors, located on the back panel layer, and TI sensors, positioned on the innermost pane of the double glass unit. Throughout the testing period, PTI sensors consistently recorded higher temperature values than TI sensors. Furthermore, within the same type of sensors, centre sensors exhibited higher values than top sensors.
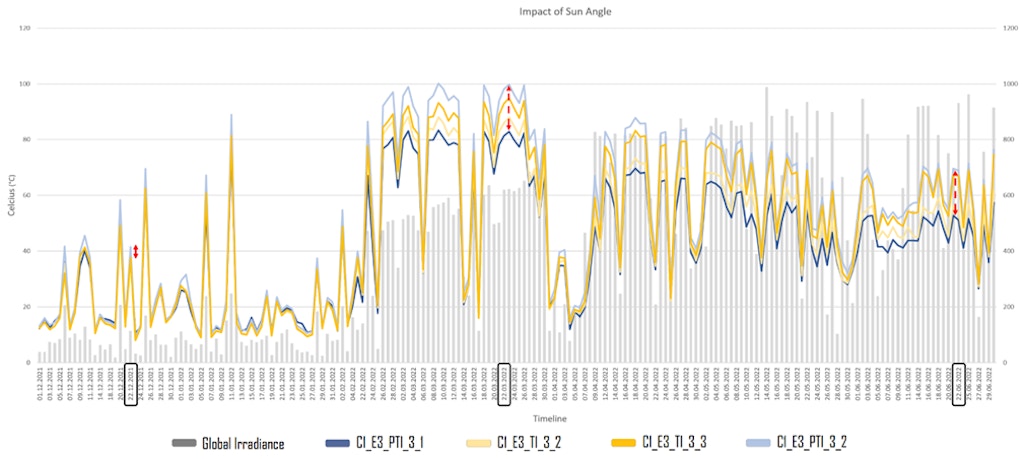
Figure 9. Impact of Sun Angle.
The analysis also revealed clear trends across different sun angles (segments), with the temperature difference between top and canter sensors being significantly higher during the summer solstice (sun angle at 66°) compared to the winter solstice (sun angle at 15°). The March equinox (sun angle at 38°) showed a relatively similar trend to the summer solstice but with smaller differences.
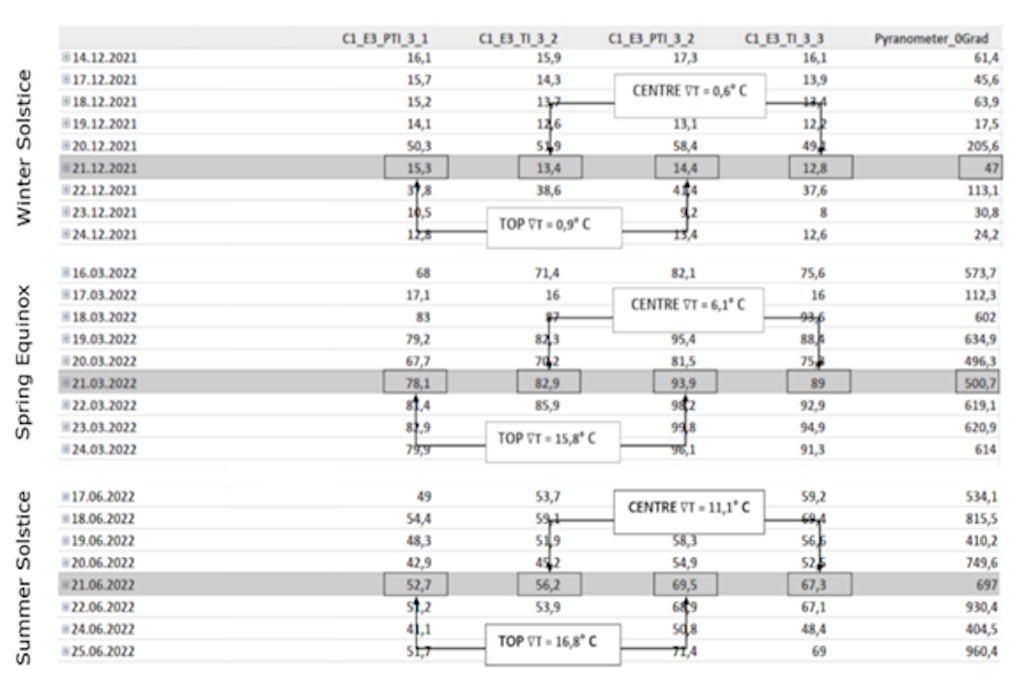
Figure 10. Data of Temperature Difference between Sensors.
The observed trends lead to a clear conclusion is that there is a positive correlation between sun angles and the temperature difference between the top and centre sensors. As sun angles increase, the temperature difference also increases. Additionally, the analysis explored the role of global irradiance in parallel to sun angle (depicted in grey), showing an upward trend similar to the temperature difference from winter to summer in 2022. However, it is noteworthy that the maximum temperature occurred in the "March equinox segment," as indicated in the previous graph.

Figure 11. Relationship between Sun Angle and Sensors Temperature.
IMPACT OF SOLAR ENERGY AND SKY-COVERAGE ON HEAT BUILD-UP OF SHADOW BOXES
In this study, the correlation between heat accumulation within the cavity and the factor of global irradiance was investigated over two consecutive days: November 18th and 19th. The prevailing weather conditions significantly influenced the observations, with November 18th characterized by rain and clouds, and November 19th featuring clear and sunny conditions. For the analysis, a Type 3 shadow box unit was utilized, incorporating measurements from a Pyranometer and TI_3_3, positioned at the centre of the innermost pane of the glass assembly.
At 12:30 on November 19th, during a day with a temperature of 0°C, yet sunny and clear, the Pyranometer recorded a value of 341.2 W/m², while the corresponding temperature recorded by TI_3_3 was 76.2°C. In contrast, on November 18th at the same time, during a day with a temperature of 9°C, yet rainy and cloudy, the Pyranometer recorded a substantially lower value of 85.9 W/m², and TI_3_3 recorded a temperature of 13.6°C. The differences between the Pyranometer readings on the two days amounted to 255 W/m², while the temperature differences recorded by TI sensors were 62.6°C. These variations emphasize the notable impact of weather conditions on the heat accumulation within the cavity.
Moreover, when integrating real-time data from the weather forecast website (figure 12), it becomes evident that the sunnier yet colder day (November 19th) exhibits higher temperature values compared to the rainy and hotter day (November 18th). This finding highlights the complex interplay between weather conditions and the thermal dynamics of the cavity.
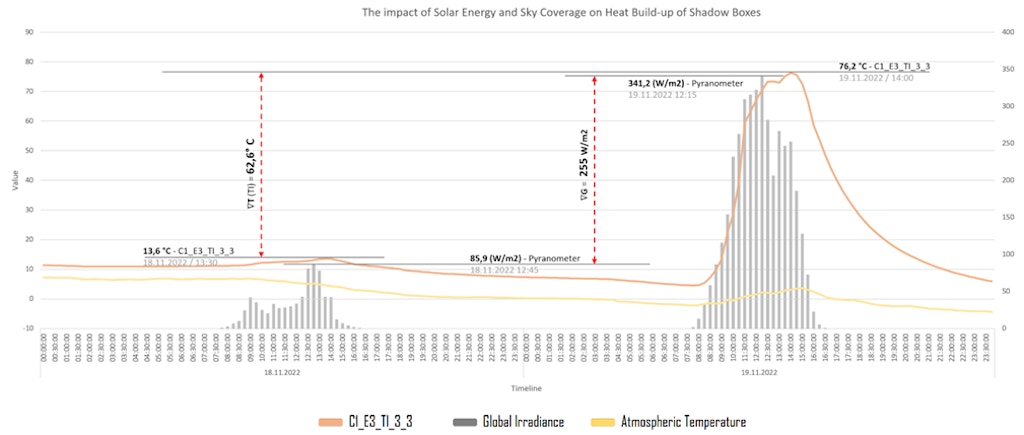
Figure 12. Impact of Solar Energy and Sky Coverage on Heat Build-up of Shadow Boxes.
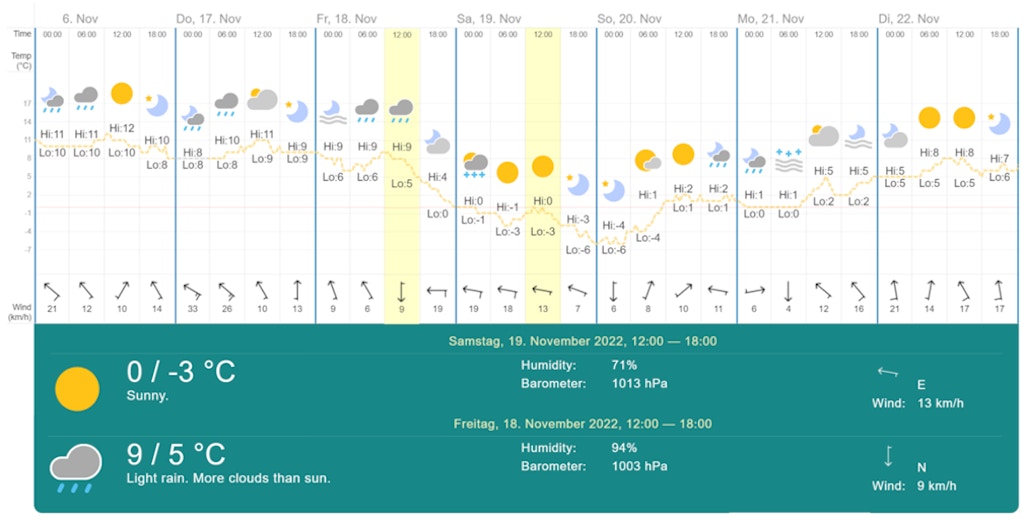
Figure 13. Real-time Weather Data, (“Sunrise and Sunset Times in Berlin, June 2022,” n.d.).
COMPARATIVE ANALSIS OF TEMPERATURE BEHAVIOUR
This chapter provides a concise yet comprehensive overview of temperature behaviors across various dimensions. Beginning with a comparison of surface temperatures during the Autumn Equinox, uncovering significant trends, notably the consistent dominance of PTI. The exploration then extends to a broader examination of PTI sensor of each type over time, highlighting key periods and the trends. Subsequently, our focus shifts to providing insights into temperature variations among different sensors, identifying benchmark values, and facilitating meaningful 1-on-1 comparisons for each sensor type.
TEMPERATURE COMPARISON OF SURFACES
This study undertakes a comparative analysis of temperature variations across different surfaces within the same unit, specifically focusing on Type 3, conducted on October 21st during the Autumn Equinox. The analysis reveals that PTI consistently demonstrates the highest temperature values, followed by TL, TI, RT, and TA in descending order. Notably, TA surpassed RT in instances where direct sun exposure occurred on the outermost glass pane, housing TA. The maximum recorded temperature was 103.6°C for PTI_3_3 at 14:00, while TA_3_1 and RT registered 51.1°C and 33.1°C, respectively. At 9 AM, all sensors initially reached the same temperature, deviating thereafter, with RT being the exception, maintaining a steady temperature while the others exhibited a rapid upward trend. As depicted in the figure on the right, an insulation board is strategically positioned between RT and PTI, effectively interrupting heat transmission from the cavity to the interior or vice versa. This phenomenon is particularly evident at 14:00 when PTI reached its peak temperature (103.6°C), while RT remained at 33.1°C. The significance of thermal separation will be elaborated upon in the forthcoming chapter on Heat Flow and Safety.
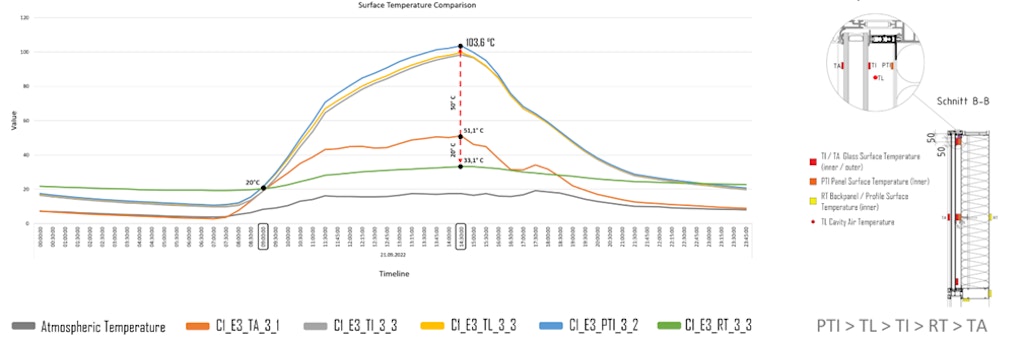
Figure 14. Surface Temperature Comparison of Sensors.
BACK LAYER TEMPERATURE BEHAVIOUR
As shown in the previous graph that PTI exhibited the highest temperature values among all types, the graph below studied the PTI sensors of all shadow box types from February, 2022 to November, 2022. As highlighted multiple times in this study, this analysis also is based upon 3 important segments, spring equinox, summer solstice, autumnal equinox.
During the equinox periods, PTI sensors exhibited temperature values ranging between 60-100°C, while during the summer solstice, temperatures fluctuated between 40-80°C. This dataset also serves as a complementary illustration to previously discussed aspects, such as the general trend of temperature and the significance of solar incident angles, in addition to the findings depicted in the earlier.
Throughout the analysis period, the PTI_4_2 sensor, represented by the green line, consistently showed higher values compared to other sensors, with PTI_3_2, PTI_2_2, and TI_1_2 following in descending order. A more in-depth analysis and comparison of each sensor's performance in two distinct months will be presented in the upcoming subchapter.
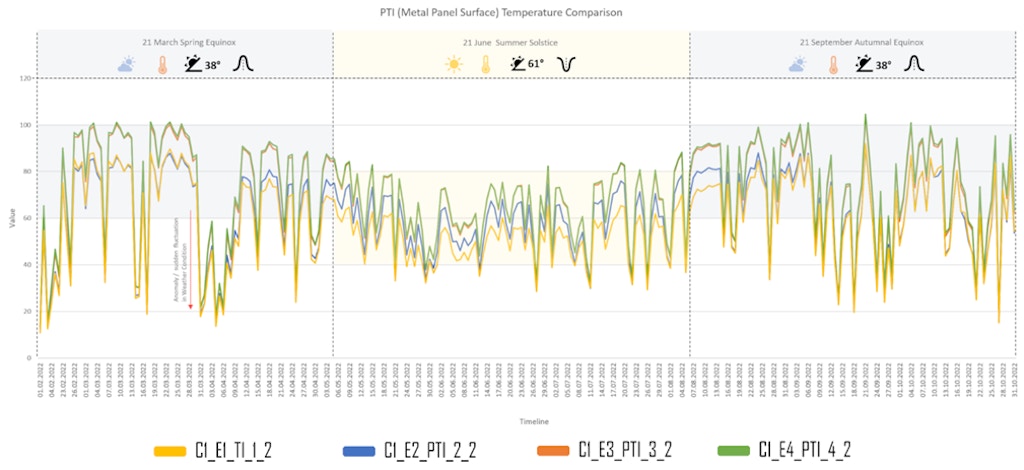
Figure 15. PTI (metal panel surface sensor) Temperature Comparison in 4 Shadow Box Units.
TEMPERATURE COMPARISON OF SENSORS
The exploration of temperature variations among PTI, TI, and TL sensors involves a comparative analysis across different shadow box types, facilitated by a excel radar charts. The analyis comprises three columns representing the sensors and two rows for the months of November and September. The sensors displayed higher values in September and lower values in November. The numerical designations 1, 2, 3, and 4 correspond to shadow box types. In November, at lower temperatures, Type 3 consistently exhibited the least favorable performance across all sensor types, with Type 2, Type 4, and Type 1 following in order from worse to better performance. Both PTI (left) and TL (right) graphs displayed a consistent pattern across all types, while the TI graph (center) highlighted the relatively distinct performance of Type 3 compared to others. At higher temperatures in September, Type 4 outperformed other types in the PTI and the TL while type 3 stood out in the TL comparison. Both PTI (left) and TL (right) graphs showed a consistent pattern across all types, while the TI graph (center) highlighted the relatively distinct performance of Type 3 compared to others. Notably, while Type 4 showed the worst performance in PTI and TL, it showcased the best performance in TI, representing the innermost pane of the glass assembly.
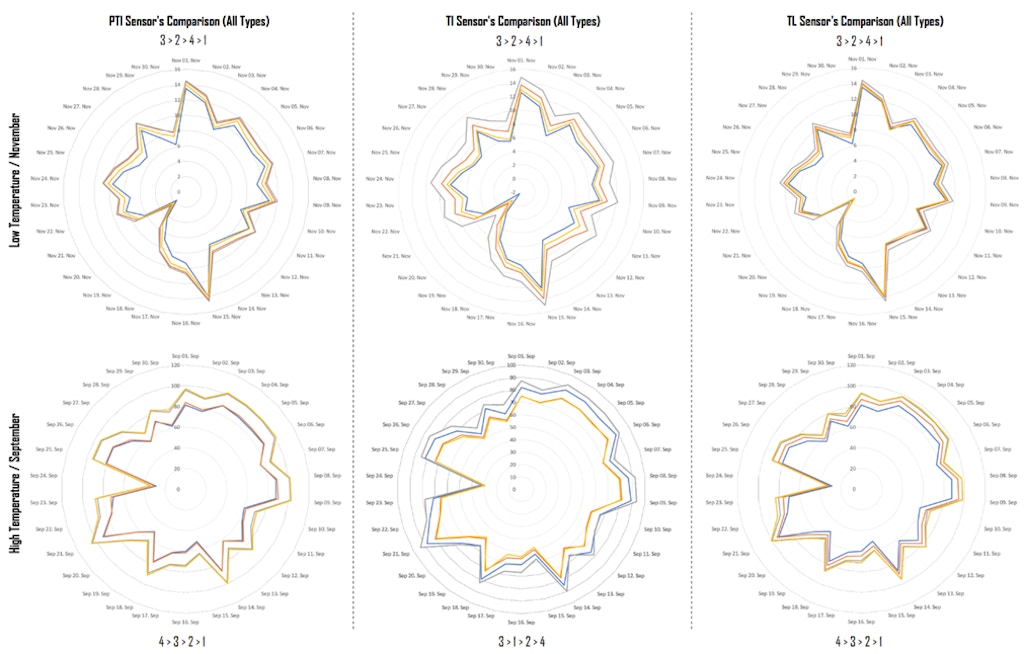
Figure 16. PTI, TI, and TL Sensors Comparison.
TEMPERATURE EXTREMES
When assessing temperature extremes, such as maximum and minimum temperatures recorded during the testing period, the graph below gives a quick overview of corresponding values. The table provides details for all types, including their respective extreme values. Additionally, the graph illustrates the number of days when TL sensor values exceeded 80°C, a critical threshold for the shadow box assembly and its components. For maximum temperature analysis, TL sensors are utilized, with Type 4 exhibiting the highest values at 100.4°C, followed by Type 3 (99.6°C), Type 2 (93.8°C), and Type 1 (92°C). Shifting to minimum recorded temperatures, TI sensors are employed, revealing Type 4 as the least performing with -2.8°C, followed by Type 1 (-2.3°C), Type 3 (-2.1°C), and Type 2 (-1.9°C). In the bottom row of the graph, the number of days above 80°C for all shadow box types in 2022 is presented, along with corresponding months and days. TL sensors are used for this analysis, excluding Type 1 due to its lack of an air cavity layer. Despite Type 4 recording the most days at 97, Type 3 closely followed with 95 days, Type 2 with 68 days, and Type 1 showing the best performance at 38 days. Examining critical months, March and August displayed the poorest performance, with Type 4 recording 24 and 21 days, Type 3 with 24 and 20 days, and Type 2 with 21 and 18 days. Notably, Type 1 stood out in March with 20 days, while August showed no such instances. Overall, these findings, in conjunction with previous observations on sun angle and its correlation with heat buildup, underscore that March and August—equinox months with a 38° sun incident angle—are when shadow boxes experienced the highest temperature values.
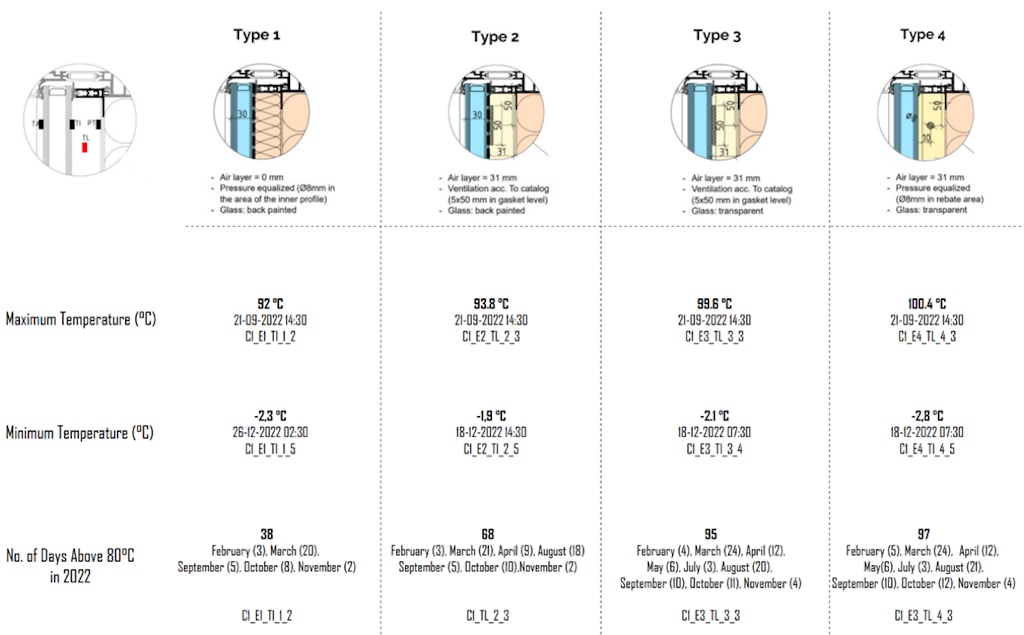
Figure 17. Overview of Temperature Results.
1-ON-1 COMPARISONS
In this analysis, we delved into one-on-one comparisons between shadow box assemblies to refine our understanding of their behavior. The previous graph presented differences in a way that made it challenging to discern correlation proximity but provided a clear view of generic differences. This graph now offers a summary of 1-on-1 comparisons, focusing on sensors and specifying numeric differences.
The first comparison features Type 3 and Type 4, conducting a detailed analysis of different sensors and temperature differences during the same time period, considering thresholds of 5°C and 10°C. Type 3 underperformed Type 4 in TL and TI center sensors, while Type 4 demonstrated superiority in PTI. Notably, in 2022 there was only one day where the temperature difference between TL_3_3 and TL_4_3 exceeded 5°C, with merely 12 days recording differences surpassing 3°C.
For the second comparison involving Type 2 and Type 3, with threshold values of 5°C and 10°C, Type 3 underperformed Type 2 in all centre sensors (TI, TL, PTI). Surprisingly, in 2022 there were only three days where the temperature difference between TL_2_3 and TL_3_3 exceeded 10°C, while 211 days exhibited differences surpassing 5°C. These variations were predominantly observed in the months of March, October, and November.
In the third comparison, featuring Type 2 and Type 4 with threshold values of 5 and 10°C, Type 4 underperformed Type 2 in TL and PTI centre sensors, while Type 2 surpassed in TI. Notably, in 2022 there were only four days where the temperature difference between TL_2_3 and TL_4_3 exceeded 10°C, with 321 days recording differences surpassing 5°C. These differences were most prominent in the months of March, April, August, September, October, and November.
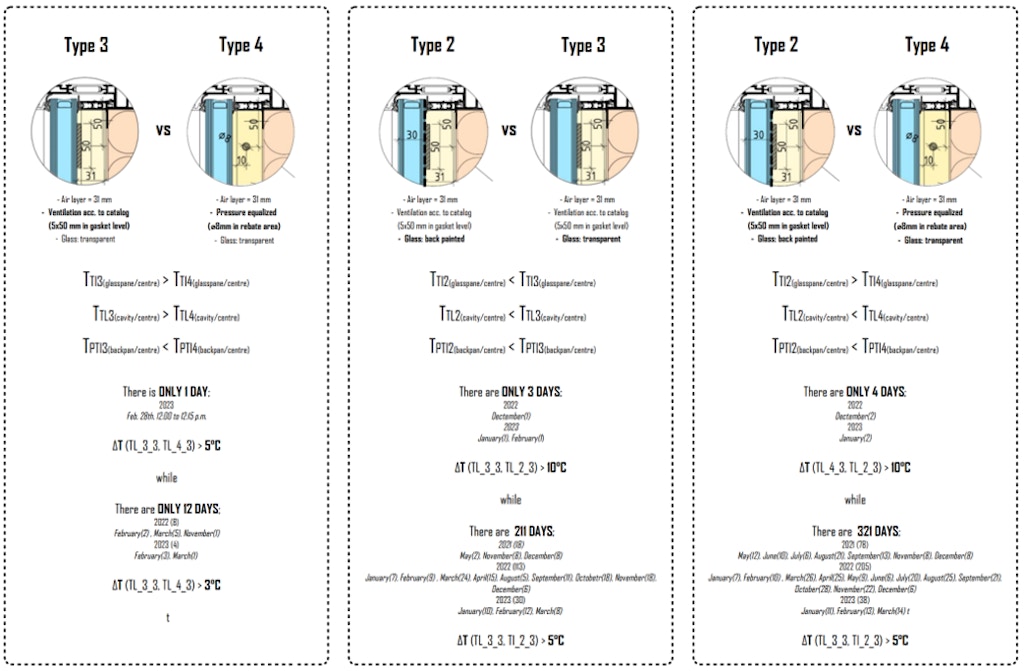
Figure 18. 1-on-1 Comparison of All Shadow Box Test Units.
HEAT FLOW AND THERMAL THERMAL BRIDGING
Examining heat flow and thermal bridging within the shadow box assembly is essential given its complex layers and adjacent structures. This analysis focuses on the Type 3 shadow box assembly during the "Spring Equinox" on September 21st, 2022. The graph highlights centre sensors, RT and TL, with a dashed red line indicating the estimated value in a hypothetical scenario without a thermal break along the shadow box perimeter. Outdoor air temperature is presented in grey columns. On September 21st at 15:00, TL_3_3 sensor reached a maximum temperature of 95.8°C, while RT_3_3 sensor recorded 34°C, marking the highest RT sensor temperature recorded during the entire period. The presence of a thermal break between the profile and the gasket behind the glass assembly significantly reduced the heat transmitted from the cavity through the inner surface of the profile by 61.8°C.
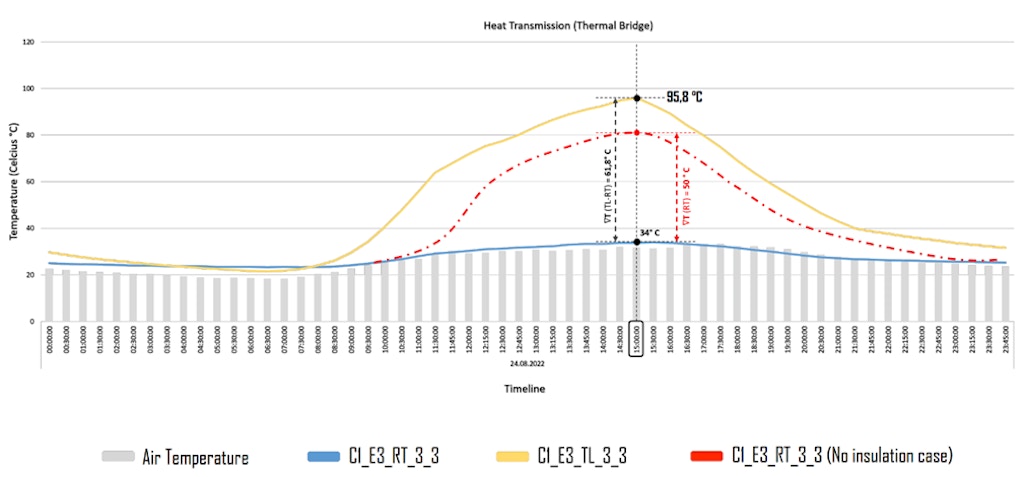
Figure 19. Heat Transmission within Shadow Box.
Artzmann (2016) notes that a thermal break between the opaque back layer and the facade transom, along with continuous insulation covering the bottom surface of the transom, results in lower temperatures on top of the transom but higher temperatures inside the shadow box. Without a thermal break, the temperature inside the cavity may be lower, but the temperature flowed inside could be at least twice as high as in the design with a thermal break. Therefore, the roughly estimated RT with the red line in the graph is considered to be around 70-80°C, indicating a potential undesired heat flow and thermal break through the shadow box to the interior.
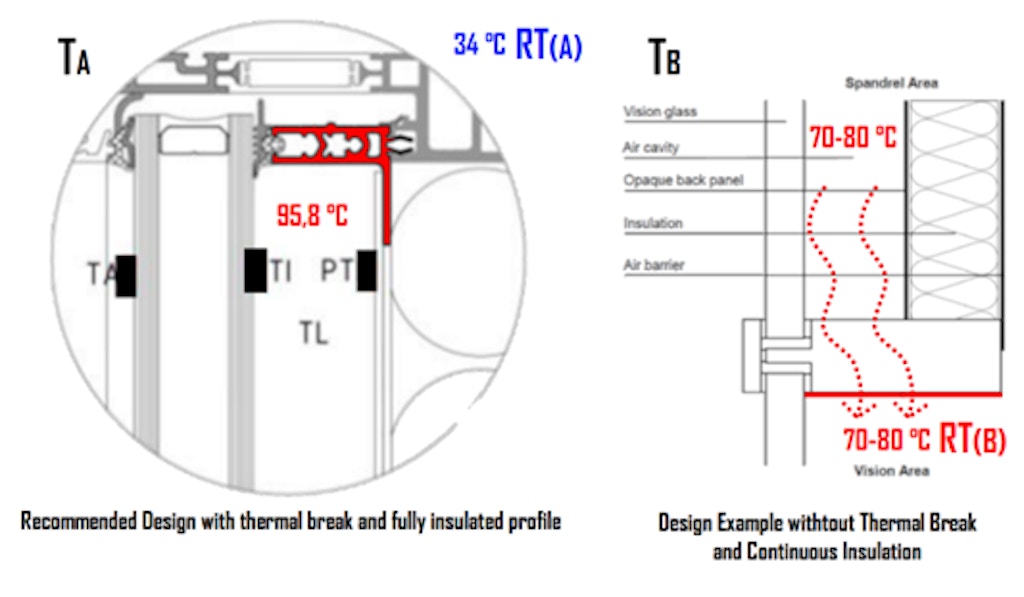
Figure 20. Importance of Thermal Break.
SAFETY AND SAFE TOUCH TEMPERATURE
Building upon the insights from the previous chapter on heat flow and thermal breaks, it's evident that the absence of properly located thermal breaks in a shadow box design results in continuous heat flow through the cavity to the interior, leading to thermal breaks and heat loss. However, the concerns extend beyond thermal loss and breaks; another critical parameter is the "safe touch temperature," which can have detrimental consequences.
On August 24th, 2022, the day when RT sensors recorded their highest values, a focused study was conducted on RT_3 sensors for all types. Type 3 exhibited the highest temperature at 34°C, followed by Type 2, Type 4, and Type 1.
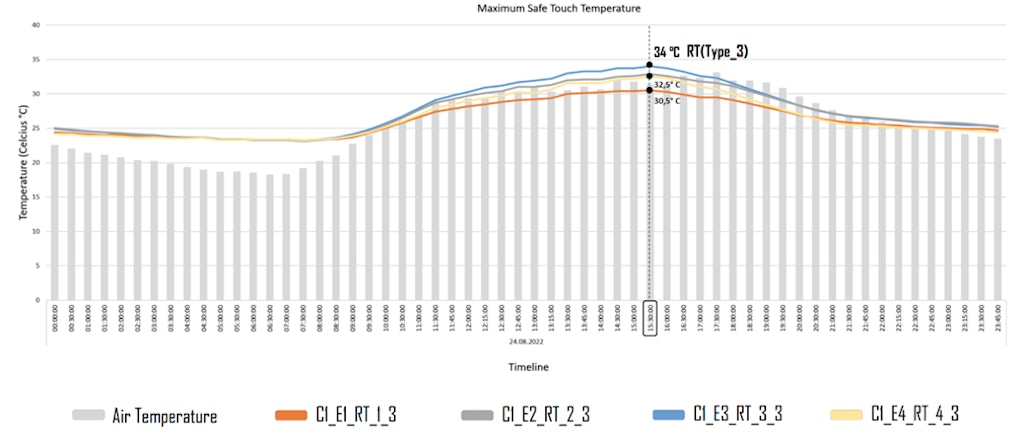
Figure 21. Maximum Safe Touch Temperature.
On the left-hand side, a graph from EN ISO 13733-1 provides a detailed representation of the burn threshold of metals concerning the contact period in seconds. It's noteworthy that the graph's non-linear nature, following an exponential pattern, emphasizes the importance of selecting the appropriate temperature value based on the specific contact duration. For example, the burn threshold for aluminium is identified as 59°C within a 5-second contact period.
In parallel, ASTM C1055 offers a table outlining pain thresholds, types of sensation, and potential injuries. By integrating data from both standards, the permissible temperature for RT is determined. The key requirement is that the maximum recorded RT must remain below the pain threshold temperature, which, in this context, is specified as 44°C.
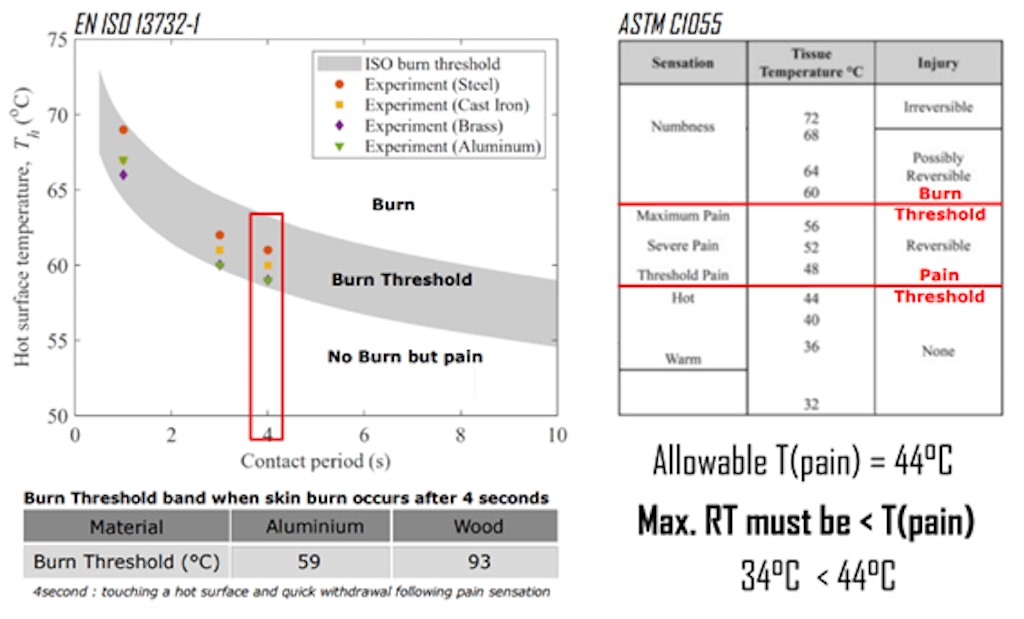
Figure 22. Service Temperature from Different Standards.
DISCUSSION
The data indicates a fluctuating temperature trend throughout the year, contradicting the idea of a consistent decrease in heat build-up in shadow box assemblies from winter to summer. Instead, the lowest temperatures are observed in winter, particularly in December, while the highest temperatures occur in March and September. This pattern can be linked to the Solar Altitude Angle, also known as the angle of the sun, and the prevailing weather conditions. The Solar Altitude Angle, also known as sun azimuth, stands out as a key factor impacting heat build-up within the shadow box cavity. Shading caused by the profile leads to fluctuating heat accumulation, with higher values when the sun angle approaches the equinox angle (38°C). The theoretical possibility of optimal heat build-up at winter solstice angles is acknowledged; however, practical constraints like sky coverage, low atmospheric temperatures, and shading from neighbouring buildings may impede the realization of this scenario in practice.
Figure 23: Left, Sun Altitude on Different Periods (“Sunrise and Sunset Times in Berlin, June 2022,” n.d.); Right; Potential Shading due to Profile Systems.
Sun incident angle, although crucial, is not the sole determinant of heat accumulation. The condition of weather, specifically the "Sky Coverage ratio" plays an integral role. Clear skies significantly impact heat accumulation, surpassing the influence of atmospheric temperature and sun angle. When sun rays fail to reach shadow box surfaces, heat accumulation in the cavity drops drastically.
Comparative analyses consistently identify Type 3 as the least performing shadow box type, followed by Type 4. In contrast, Types 2 and 1 consistently outperformed others, showcasing the positive impact of opacifiers and the absence of an air layer (substituted with insulation) on heat build-up. The sensor analysis revealed elevated PTI readings compared to other sensors, attributed to its placement on a metal back pan, which tends to accumulate heat more than other surfaces within the shadow box.
To ensure accurate data interpretation, an in-depth sensors comparison was undertaken, focusing specifically on day-based data. Despite initial graph impressions, the one-on-one comparative analysis showed that the recorded temperature difference between type 3 and type 4 is less significant. In 2022, only four days exhibited a temperature difference exceeding 5°C simultaneously, indicating their similar behaviour. Conversely, comparing type 2 with type 3 or type 4 revealed distinct temperature difference patterns throughout the year.
As mentioned in the corresponding chapter, safe touch temperature, the surface temperature of profile, must be below the pain threshold specified by the international or local regulations. This consideration is crucial to align with safety standards and prevent any discomfort or injury associated with elevated temperatures.
Additionally, it is important to understand the limits of materials when considering laminated glass due to the presence of a PVB interlayer. When temperatures reach up to 100°C, it's crucial to recognize that PVB interlayers can only resist up to about 80°C. Thus, selecting tempered glass and understanding the possible complications with laminated glass interlayers are key measures to ensure the durability and safety of glass components in the shadow box assembly.
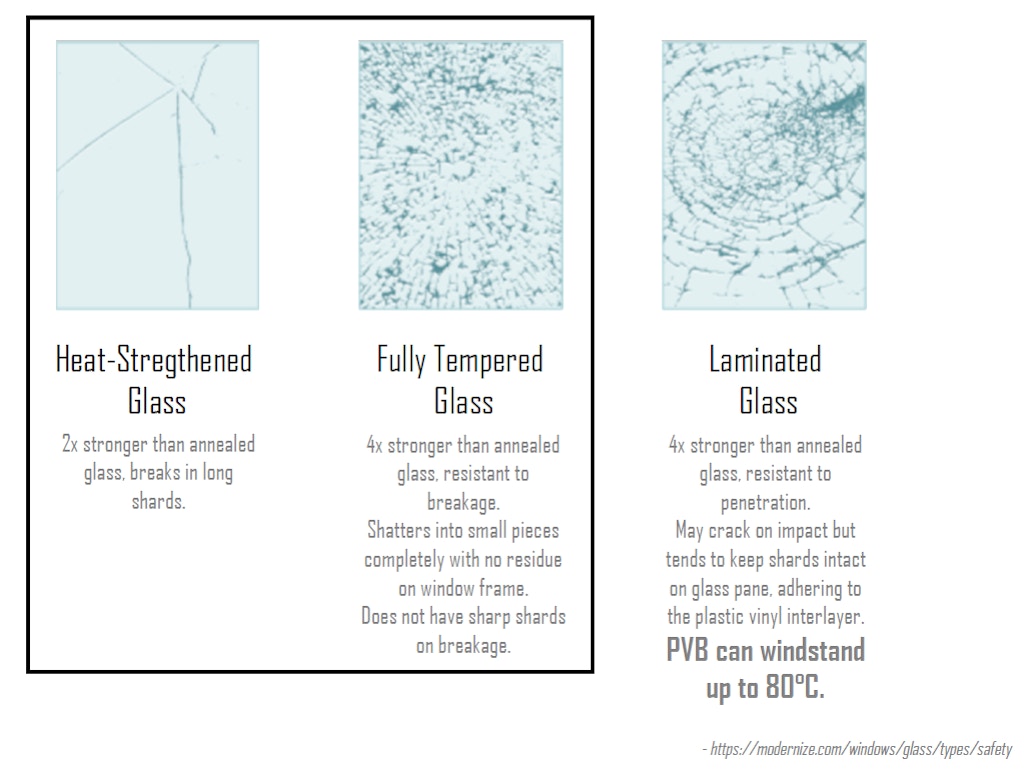
Figure 24: The Importance of Temperature on Glass Selection.
CONCLUSION AND FUTURE WORK
This paper addresses the issue of overheating in Shadow Box Façade Systems through a mock-up test approach. A significant contribution of our work is the in-depth comparison of four commonly used shadow box assemblies. This comparison provides valuable insights into further understanding of the complex thermal behaviour of shadow box systems. This follow-up study reinforces the initial study that the incident angle of the sun plays a predominant role in influencing heat build-up within shadow boxes, surpassing the impact of solar radiation intensity. However, it's crucial to recognize the comparable importance of prevailing weather conditions, especially during overcast skies. In such conditions, sun rays are obstructed, preventing them from reaching the shadow box and resulting in less heat accumulation inside the cavity compared to clear sky conditions. These findings clearly explain why high temperature values observed during the months of March, April, September, and October where there is a higher occurrence of clear sky days with a low sun angle.
This study has several implications for the design and construction of shadow box systems. Given that heat development in shadow boxes is influenced by various stimuli, primarily dominated by sun angle and sky clearance, designers should consider these external variations when determining the orientation and placement of the shadow box. However, it's also crucial to acknowledge that these four types do not encompass the full spectrum of shadow boxes available in the market. Consequently, the findings from our research should not be directly generalized as a reference for other shadow box applications, especially those in different climates and settings.
Furthermore, considering the impact of neighbouring buildings and their shading effects on shadow boxes presents a promising area for future research. Developing strategies to mitigate these external factors and improve the predictability of heat build-up in different environmental conditions could significantly enhance the reliability of shadow box applications. Additionally, expanding the study to real-world applications and monitoring shadow box systems in operational buildings over an extended period could provide invaluable data. This longitudinal approach would offer a more comprehensive understanding of the long-term performance and durability of shadow box assemblies across diverse climates.
In conclusion, the ultimate goal is to provide valuable insights to all stakeholders, contributing to the establishment of a consensus on shadow box design and construction within the industry. Achieving this objective requires strong collaboration among architects, engineers, and researchers, coupled with a deep awareness of shadow box dynamics. This collective effort is essential to develop standardized guidelines. Thus, this research serves as a foundational step toward fostering a more informed and sustainable approach to the integration of shadow box assemblies in modern glazing facades.
RECOMMENDATIONS
The application of a shadow box itself is susceptible to distinct problems, given the complex nature of its thermal dynamics. This complexity necessitates careful design using appropriate materials. Therefore, it is important to understand the behaviour of the shadow box as a system and evaluate the performance of each application. Recognizing the impracticality of creating mock-ups for every project, a strong recommendation is to conduct 2D and 3D analyses. Drawing insights from studies such as ours to determine the variables can significantly contribute to identifying the most effective project-specific design approach.
Thermal breaks for a shadow box assembly are as important as other elements such as the glass unit and insulation. In cases where no thermal break is used, this can lead to various problems such as exceeding the safe touch temperature, which can have detrimental effects on users and result in a decrease in the overall thermal efficiency of the building, accompanied by an increase in cooling and heating loads. Therefore, it is strongly recommended to incorporate a thermal break—effectively disrupting the heat flow between the room side and the cavity—into the design for optimal performance.
To address the off-gassing problem, it is essential to explore different materials and incorporate sensors capable of measuring air ratios within the cavity. This approach allows for the evaluation of material suitability, particularly in scenarios where temperatures can reach up to 105°C. Understanding the potential adverse effects of off-gassing is critical, as it may lead to chemical gas emissions and visual distortions such as the Moiré effect. Therefore, selecting the right material for shadow boxes, especially in hot climates, becomes vital to effectively mitigate these risks.
Given the potential for temperatures to reach up to 100°C, it is critical to consider the thermal tolerance of materials used in shadow box assemblies. Polyvinyl butyral (PVB) interlayers, commonly employed for lamination process of laminated glass, have a resistance limit of approximately 80°C. Exceeding this temperature can compromise the interlayer's integrity and effectiveness. Therefore, it is recommended to opt for tempered glass when designing shadow box assemblies.
Acknowledgements
We express our gratitude to everyone who provided valuable comments and insights during the development of this research document. Special acknowledgment goes to AGC Glass Germany GmbH and Schüco International KG for their generous research funding, which significantly contributed to the completion of this project.
We also extend our sincere thanks to Godo Zabur Singh and Alvaro Balderema, whose pivotal roles in the initial study laid the foundation for this follow-up research. Their contributions have played a crucial role in shaping the direction and success of our work.
Rights and Permissions
Arztmann, Daniel. 2016. "Shadow Boxes – Re-Engineered: results from technical research and project design." Facade Tectonics Institute (Eds.), Facade Tectonics : World Congress Los Angeles 2016 Conference Proceedings, 2. Los Angeles: Tectonic Press. 10. https://www.facadetectonics.org/publications/collection/publications.
ASTM. 2020. “Standard Guide for Heated System Surface Conditions that Produce Contact Burn Injuries.” ASTM C1055-20, ASTM International, West Conshohocken, PA. DOI: 10.1520/C1055-20.
Bauer, Max, Armin Bäumler, and Martin Bauer. 2015. “Hinterlüftung Und Tauwasserausfall Bei Verbundfenstern.” Bauphysik 37 (4): 223–28. https://doi.org/10.1002/bapi.201510024.
Boswell, Keith and John Walker. 2005. "Shadow Boxes - An Architect and Cladding Designers’ Search for Solutions". Glass Processing Days 2005: Proceedings of the 9th International Conference, Tampere, Finland.
Brzezicki, Marcin. 2014. "Redundant transparency: The building's light-permeable disguise." Journal of Architectural and Planning Research.
Charles Pankow Foundation. 2023. "Thermal Performance of Spandrel assemblies in glazed wall systems." Phase 1: Design Test Program Final Report, Haymarket, VA, USA. https://www.pankowfoundation.org/site/assets/files/2320/04-22_thermal_performance_of_spandrel_assemblies_in_glazed_wall_systems-1.pdf.
Cucuzzella, Carmela, Negarsadat Rahimi, and Aristofanis Soulikias. 2023. "The Evolution of the Architectural Façade since 1950: A Contemporary Categorization" Architecture 3, no. 1: 1-32. https://doi.org/10.3390/architecture3010001.
CWCT. 2014. "Technical Note No.94 - Shadow Boxes." Centre for Window and Cladding Technology. https://www.cwct.co.uk/products/technical-note-94.
EN ISO. 2006. “Ergonomics of the thermal environment - Methods for the assessment of human responses to contact with surfaces - Part 1: Hot surfaces” EN ISO 13732-1:2006, ASTM International, BSI, Brussels, BE. DOI: https://dx.doi.org/10.31030/1471484.
Haldimann, Matthias, Andreas Luible, and Mauro Overend. 2008. “Structural Use of Glass.” International Association for Bridge and Structural Engineering IABSE, January. https://doi.org/10.2749/sed010.
Kragh, Mikkel. 2014. "Performance of Shadow Boxes in Curtain Wall Assemblies." CTBUH 2014 Shanghai Conference Proceedings. 6.
Lang, Andy. 2010. "Design Considerations for Shadow Boxes in Curtain Wall Glazing." ICBEST 2010 Conference Proceedings, 2.
McCowan, Derek, Mark Brown, and Micheal Louis. 2008. “Curtain-Wall Designs.” Glass Magazine. May 28, 2008. https://www.glassmagazine.com/article/curtain-wall-designs.
Murray, Scott Charles. 2009. Contemporary Curtain Wall Architecture. 1st ed. New York: Princeton Architectural Press.
Pilkington. 2012. "Technical Bulletin - Spandrel Panel Glazing ATS-124." ATS-124, Pilkington North America Inc., Toledo, OH, USA. https://www.pilkington.com/en/us/window-manufacturers/technical-bulletins#
Saint-Gobain. 2018. "Thermal Safety 3A: The resistance of glass to thermal Stress" Saint-Gobain Building Glass, UK.
Schwartz, Julien, Patrick Roppel, Stephane Hoffman, Neil Norris.. 2017. " Quantifying The Benefit of Venting Glazed Spandrels to Reduce Glass Breakage and Control Moisture." 15th Canadian Conference on Building Science and Technology Proceedings, Vancouver, BC, Canada.
Singh, Godo Zabur, Daniel Arztmann, and Alvaro Balderrama. 2023. “Investigating Heat Development in Shadow Box Façade Systems: A Mockup Test Approach.” ISCCGF- Zagreb 2023 Conference Proceedings. University of Zagreb, Faculty of Architecture. Zagreb, Croatia.
“Sunrise and Sunset Times in Berlin, June 2022.” n.d. https://www.timeanddate.com/sun/germany/bielefeld?month=6&year=2022.
“Thermal Stress: Film-to-Glass Compatibility.” 2012. EWFA. November 14, 2012. https://www.glassonweb.com/article/thermal-stress-film-glass-compatibility.
Walsh, Mark. 2018. "To Vent Or Not To Vent." Facade Tectonics Institute (Eds.), Facade Tectonics : World Congress Los Angeles 2018 Conference Proceedings, 2. Los Angeles: Tectonic Press. 10. https://www.facadetectonics.org/publications/collection/publications.