Dynamic Shading Device
Development of a Luminous and Thermal Responsive Brise-Soleil
Presented on October 9, 2024 at Facade Tectonics 2024 World Congress
Sign in and Register
Create an Account
Overview
Abstract
Responsive shading systems can dynamically improve indoor environmental conditions based on outdoor climate variations. Among the technologies, low-cost microprocessors and sensors associated with computational algorithms control the performance of pre-programmed tasks such as the opening, closing, and rotation of elements integrated into the façade. This paper presents the design, construction, and performance evaluation of a responsive brise-soleil constructed in Curitiba, PR, Brazil (Cfb, humid subtropical). This project is part of an ongoing Ph.D. thesis. The system is based on a small-scale model developed at Lyle Center for Regenerative Studies during the winter season in California, USA. The responsive device functionality considers luminous and thermal comfort parameters: Useful Daylight Illuminance and the Adaptive Comfort Model (ANSI/ASHRAE 55). This device adapts the regular use of a commercial brise-soleil louver and motorization system into upward and downward direction slat rotation regulating direct and diffuse solar radiation incidence. The window is subject to three shading patterns: 0% shade, 50% shade, and 100% shade. The responsive rules consider outdoor air temperature, indoor air temperature, globe temperature, operative temperature, neutral operative temperature, indoor illuminance at the work plane, and the current solar angle and azimuth. Preliminary results of the full-scale model applied on the façade of a Cost-effective Bioclimatic Building Chamber (CBBC) demonstrate the system's feasibility in controlling indoor air temperature and illuminance at the work plane. The performance evaluations considered two scenarios: statically and responsively shaded. The responsive device reduced the period when the operative temperature remained under the lower thermal comfort threshold and prevented overheating, reducing the demand for heating and cooling systems. The period when the illuminance level remained within the comfort level was proportional for both scenarios. However, the responsive system reduced the amount of time when the user would be subject to discomfort caused by glare. This responsive device concept is subject to further improvements and implementation on buildings, contributing to reducing energy demand during the operational phase for lighting, cooling, or heating. The design concept and functionality criteria might be interesting for dynamic façade developers.
Authors
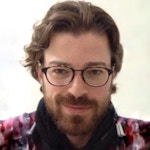
Gabriel de Bem
Professor
gabrieldebem@gmail.com
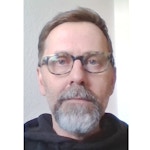
Eduardo Krüger
Professor
ekruger@utfpr.edu.br
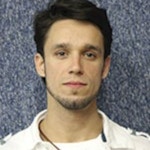
Alexandre Augusto Alberto Moreira de Abreu
Professor
alexandre.abreu@ifsc.edu.br
Keywords
Paper content
Introduction
Architecture and construction are undergoing rapid transformations, supporting the development of both static and dynamic constructive systems[1]. In the design stage, the consideration of a given building's adaptability to evolving functions over its life cycle is often overlooked [2]. In addition to contemplating spatial utility and user dynamics, there is the need to account for potential changes in momentary climatic conditions. Building projects based on historical weather data can not anticipate future shifts in urban landscapes and climate, particularly considering the current climate crisis. Technological advancements have facilitated the development of buildings with kinetic elements, providing them with flexibility and adaptability [2] [3].
In the domain of responsive façades, Bem et al. (2022) [4] have devised a simple system that dynamically adjusts shades on a test cell based on the sun's position. The efficacy of this system was experimentally tested as a pilot study with a small test cell in Pomona, California, a location characterized by a Mediterranean climate with hot summers, Csb [5]. This study extends its initial findings, implementing analogous methods in a full-scale test environment. The experiment was developed in Curitiba, a temperate oceanic climate with mild summers (Cfb) [5]. Herein, we present insights derived from the comparative analysis of two scenarios: one employing fixed shading elements (traditional brise-soleil) and the other with the window responsively shaded.
Method
The responsive system's functionality is grounded in the Adaptive Comfort Model outlined in ANSI/ASHRAE 55 [6], where a maximum daily thermal variation of 5°C from the Neutral Temperature (Tn) corresponds to 90% user acceptability. Real-time thermal recording aligns with the Operative Temperature (To) calculation specified in the same standard. Lighting parameters for the office work plane follow limits established by the Useful Daylight Illuminance metric, UDI [7], with a variation range between 100 and 2000 lx.
The test environment measures 3.00m x 2.44m x 2.89m (l x w x h). A single facade has a 6mm tempered glass window (1.40m x 0.90m). The window-to-wall ratio (WWR) is 24%. The envelope (an adapted cargo container-type building) consists of a corten steel sheet (2mm), sprayed expanded polyurethane (15mm), OSB sheet (11.1mm), wall panel (55mm), and plasterboard finish (12.5mm), resulting in a thermal transmittance (U) of 0.869 W/m².K. The floor comprises expanded polyurethane (15mm), naval wood flooring (28mm), wall panel sheet (55mm), and vinyl floor finish (3mm), with a total thermal transmittance of 0.997 W/m².K [9].
The system uses cost-effective devices, with a Raspberry Pi microprocessor managing the responsive behavior, programmed with Python programming language version 3.9.5. Data is stored locally in a relational database, sqlite3. An independent and redundant mechanism increases data storage safety, allowing real-time access to them and performance evaluation far from the experimental site. The test environment and sensor distribution are illustrated in Figure 1.
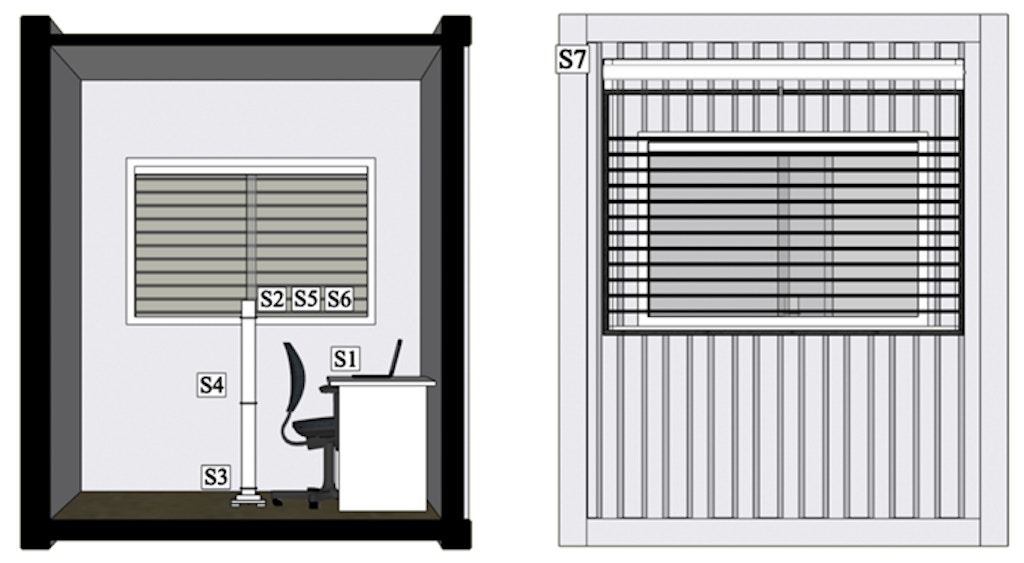
Figure 1 - Test environment and sensor distribution
Lighting levels are recorded by two BH1750FVI sensors: S1 (Etable), installed on the table (0.75m), and S2 (Eeye), positioned at a height of 1.10m facing the wall, equivalent to the eyes of a seated user. Five DS18B20 digital temperature sensors collect thermal data. Following ISO 7726:1985 guidelines [8], sensors are positioned at various heights: S3 (Tfoot, 0.10m), S4 (Tabd, 0.60m), S5 (Thead, 1.10m) for indoor air temperature (Tin) determination. At the same height (1.10m), S6 measures globe temperature (Tg) for mean radiant temperature (Tmrt) calculation. On the facade, S7 (Tout) is positioned to measure the outdoor air temperature (Tout) to determine the prevailing mean outdoor air temperature (Tpm) and neutral operative temperature (Tn).
The responsive system uses thermal and lighting data to generate three shading patterns based on trigonometric equations presented in [10], with adjustments accounting for solar azimuth. These patterns aim to optimize the balance between natural lighting and thermal comfort indoors: 0% shading allows for the maximum incidence of direct solar radiation into the environment; 50% shading adopts a rotation angle for half of the window area under direct sunlight, and 100% shading provides total shading for the window.
The analysis involves two comparative studies: window shaded by a static brise-soleil and shaded by the responsive system. These comparative studies aim to provide insights into the effectiveness of the responsive shading system compared to a static shading solution. The goal is to assess how adaptability to environmental conditions enhances overall performance and the maintenance of thermal and luminous comfort parameters.
Preliminary results
The results presented correspond to the winter season, focusing on the simulation of shading patterns developed in SketchUp software and data collection encompassing static shading (01-06 July) and responsive shading (22-27 July).
Figure 2 displays simulations developed for June 21, with the CBBC facade facing north at noon. The shading patterns include 0% (A, θ = -41,07°), 50% (B, θ = -18,92°), and 100% (C, θ = 7,86°). These rotation angles of the brise-soleil slats were derived from the trigonometric equations based on the sun’s position, as detailed in the methodology section.
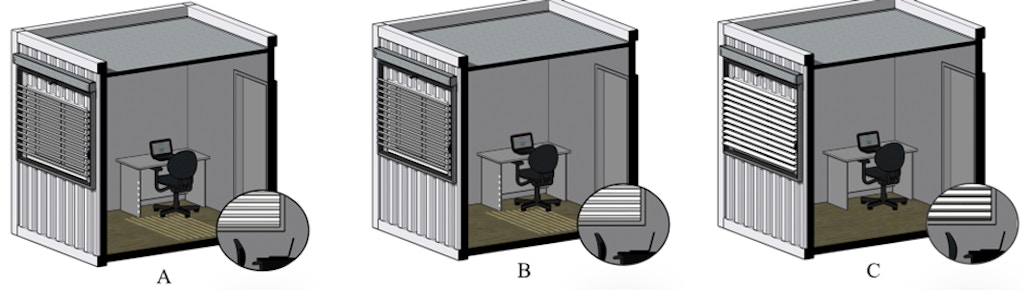
Figure 2 - Shading simulation for June 21st, at noon.
The simulation represents distinct outcomes based on the operative temperature (To) and illuminance levels concerning the comfort range, providing valuable insights into the performance of the shading patterns.
0% Shading Pattern:
When To is below the comfort range (To < Tn - 2.5°C), the 0% shading pattern permits a significant gain in direct solar radiation. In this scenario, solar incidence covers an area equivalent to the projection resulting from the window, excluding the shading generated by the thickness of the slats. Consequently, a larger area of the floor and walls is exposed to solar heat gain.
50% Shading Pattern:
The second shading pattern (50%) occurs when the To value aligns with the adaptive comfort range. Here, thermal gain is tolerable, and luminous gain is desirable. This results in a reduction of the area exposed to direct or diffuse radiation by 50%.
100% Shading Pattern:
Upon reaching the upper limit of the comfort range (Tn + 2.5°C), the brise-soleil blocks the incidence of direct or diffuse solar radiation by rotating or closing the fins with an angle (θ) corresponding to the maximum rotation angle. This action reduces both thermal and luminous gain due to direct and diffuse radiation. In the simulation presented in Figure 2 C, due to the solar height, 100% shading is achieved without the rotation of the slats, like a static brise-soleil.
Regardless of the shading pattern adopted, the rotation angles result in certain façade permeability, allowing users to interact with the outdoor environment.
Luminous performance with static shading
Data collection with static brise-soleil occurred from July 1 to 6, and Figure 3 displays the recorded illuminance levels from 07:00 AM to 06:00 PM. Black dash-dot-dot lines separate the days. Green and red lines represent the lower and upper comfort limits (100 lx and 2000 lx, respectively). The blue line corresponds to light levels on the work plane, and the gray lines represent eye-level illuminance for a seated user.
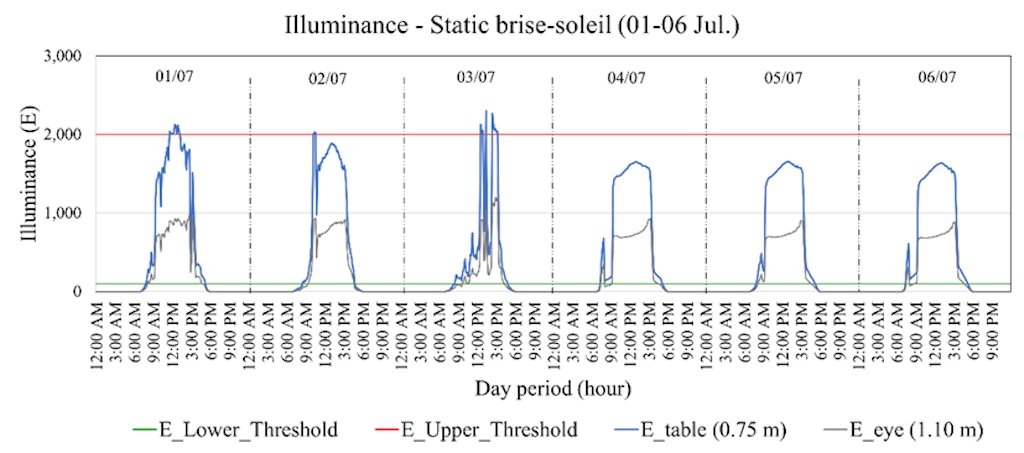
Figure 3 - Illuminance performance - Static brise-soleil
Records with Etable below the desired minimum (E < 100 lx) occur during early morning and late afternoon due to natural light availability. Lighting levels mostly stay within the comfort range (100 lx ≤ E ≤ 2000 lx), becoming more significant in the last three days due to lower cloudiness conditions. However, static brise-soleil control proves insufficient in the first three days, surpassing the maximum comfort limit (E > 2000 lx) in 24 records. This persists until external illuminance reduces due to increased cloudiness or solar movement, resulting in shading by the static brise-soleil. Notably, the illuminance at eye level remains below the maximum acceptable limit of 2000 lx.
Thermal performance with static shading
Figure 4 illustrates the thermal record, with black dashed lines separating the days. Green and red lines represent the lower and upper comfort limits (Tn – 2.5°C and Tn + 2.5°C). The blue line denotes the operative temperature (To), and the gray one represents temperature recordings at different heights (feet, abdomen, head), allowing the temperature stratification analysis. A gray dashed line represents outdoor air temperature (Tout).
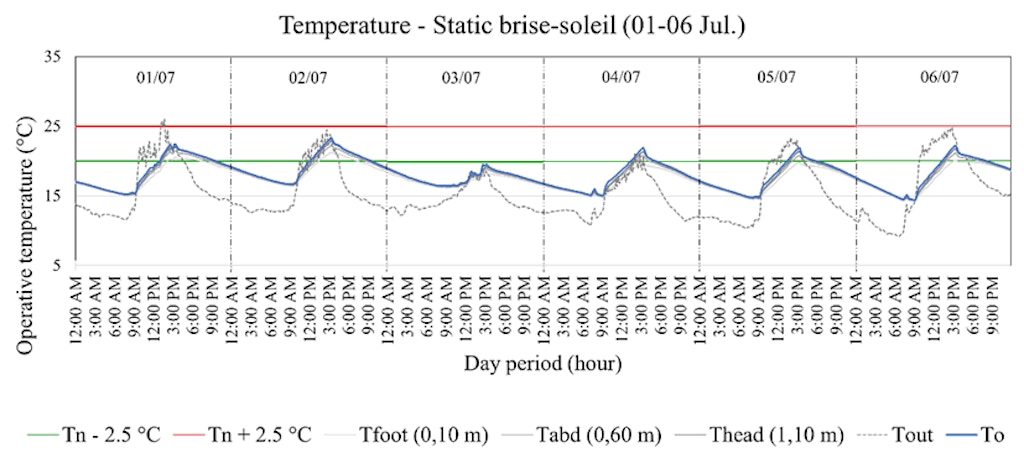
Figure 4 - Thermal performance - Static brise-soleil
The thermal performance of static brise-soleil is more influenced by outdoor air temperature variations, balancing the thermal conditions between the internal and external environments. As it partially blocks direct solar radiation, the lower comfort limit is reached only in the afternoon, except for 07/03, when records stayed below the lower comfort limit throughout the day. To never exceed the upper comfort limit, consistent with Tout variations mostly staying below this limit. Regarding thermal stratification, the maximum difference between Tfoot and Thead was 1.63°C at 03:20 PM on day 2, indicating no significant thermal discomfort.
Lighting performance with responsive shading
Data collection with the responsive shading system occurred between July 22-27. Figure 5 shows the illuminance behavior from 7:00 AM to 6:00 PM. The line representation criterion follows the same parameter adopted for the static system.
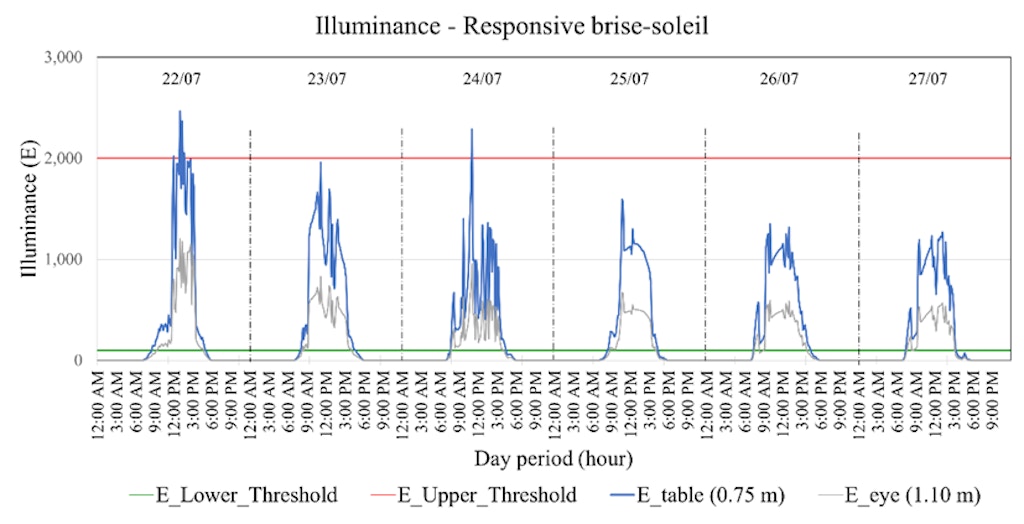
Figure 5 - Illuminance performance - Responsive brise-soleil
By managing light gains at acceptable comfort levels (100 lx < E < 2000 lx), the responsive system maintained proper conditions for carrying out office activities during at least 70% of the period measured over the six days. In turn, discomfort conditions caused by excessive illuminance on the work surface were observed on two days. During the 22/07, four moments were recorded in which the light incidence exceeded the upper limit of the comfort range (E > 2000 lx), and on the 24th, just once.
Thermal performance with responsive shading
Figure 6 illustrates the thermal performance of the responsive brise-soleil, maintaining the same line representation patterns used previously.
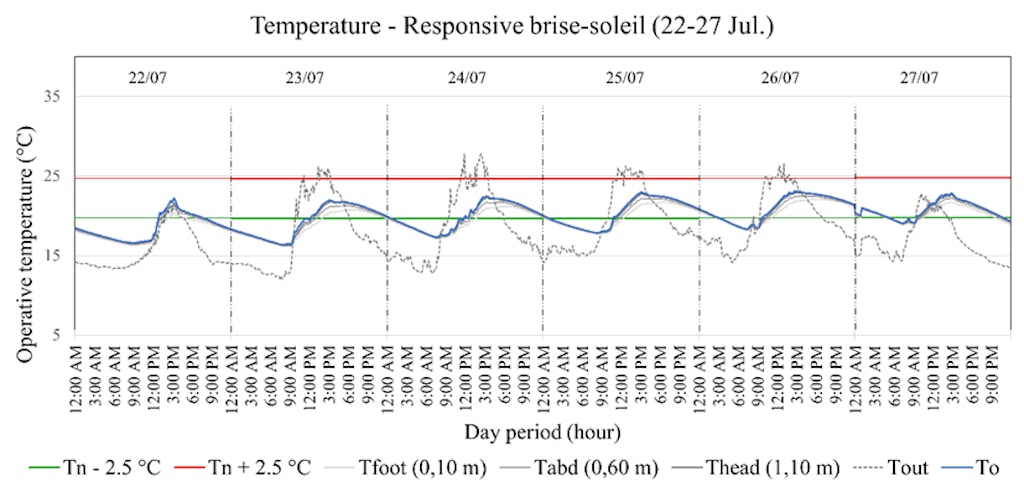
Figure 6 - Thermal performance - Responsive brise-soleil
The dynamic shading provided by the responsive brise-soleil significantly reduces the exposure period for occupants to discomfort caused by cold conditions (To < Tn – 2.5°C). Throughout the six days of data collection, To never reached overheating levels (To > Tn + 2.5°C), although Tout's records exceeded this threshold on four out of the six days (07/23 – 07/26). Like the observations during static shading, the thermal stratification resulting from direct sunlight would not bring about thermal discomfort to a seated occupant. Notably, during this data collection, To was closer to the thermal comfort range at the beginning of the day than the previous series (static brise-soleil), enhancing the overall thermal comfort provided by the responsive shading system.
Conclusion
Based on the results obtained from the responsive system, it emerges as a superior solution for mitigating discomfort caused by cold and overheating. The dynamic shading not only optimizes thermal comfort by reducing exposure to cold conditions (To < Tn – 2.5°C) but also effectively prevents overheating, as To never surpassed the upper comfort limit (To > Tn + 2.5°C). Regarding lighting control, the dynamic shading system significantly diminishes periods of user discomfort caused by excessive illuminance (2000 lx). In addition, the simplified geometry associated with low-cost responsive-processing components facilitates its implementation with small budgets.
Due to dynamic behavior, responsive devices tend to be viable and effective solutions for addressing climate variations resulting from cold spells in summer and heat waves in winter.
The simple geometry associated with low-cost sensors and microprocessors emerges as a practical solution for managing the functionality of dynamic facade construction systems, offering both efficiency and affordability. This responsive element also provides a certain level of facade permeability while shading. The outdoor visibility allows the creation of essential references for occupants, such as elapsed time, climatic conditions, location, and security. The possibility of focusing on outdoor targets also contributes to eye health. Another advantage relies on the illuminance and thermal control performed within comfort parameters without user intervention, being helpful for open-plan office layouts, where personalized adjustments are not allowed.
The system could also operate at night, contributing to the window insulation when fully closed or redirecting nighttime ventilation. During rainy days, water is prevented from splattering indoors, enabling façade permeability. Incorporating an anemometer and a rain detector sensor at the façade would further improve such functions. Adopting a concave shape for the upper located brise-soleil slats might improve the light reflection to the ceiling and contribute to indoor luminous distribution. Apart from the building performance, adopting colored or silk-screened surfaces would produce a performative and artistic element to the facade.
It is essential to note that the two series were subject to notable variations due to factors such as cloudiness and outdoor air temperature fluctuations. This natural behavior highlights the relevance of dynamic shading in accommodating sudden temperature variations even in the same season. For a more equitable assessment between the two systems, future studies should be conducted under similar environmental conditions, encompassing maximum and minimum Tout weather forecasts and To at the beginning of the day from indoor thermal preconditioning.
This consideration underscores the need for further research to comprehensively evaluate the performance of this dynamic shading device in diverse environmental contexts. The upcoming steps will also include the user’s thermal and luminous perception. Such studies will contribute to a more robust understanding of the system's effectiveness and applicability.
Acknowledgements
We want to thank Sun Fix and Somfy suppliers for all the support provided.
Rights and Permissions
[1] T. V. Belyaeva, "Dynamic architecture: new style forming aspects.," in International Conference on Construction, Architecture and Technosphere Safety (ICCATS 2017), Chelyabinsk, 2017.
[2] H. Achten, "Interaction Narratives for Responsive Architecture," Buildings, vol. 9, no. 66, p. 11, 2019.
[3] A. Desai, Designing building skins, Cambridge: Massachusetts Institute of Technology, 1992.
[4] G. d. Bem, P. La Roche, E. L. Krüger and A. A. A. M. d. Abreu, "Responsive brise-soleil: design concept and performance analysis," in Passive and Low Energy Architecture: Will Cities Survive?, Santiago, 2022.
[5] G. Betti, F. Tartarini, C. Nguyen and S. Schiavon, CBE Clima Tool: a free and open-source web application for climate analysis tailored to sustainable building design, Berkeley, 2022.
[6] R. a. A. C. E. American Society of Heating, ASHRAE Standard 55-2020, Atlanta: ASHRAE, 2020.
[7] A. Nabil and J. Mardaljevic, "Useful daylight illuminance: a new paradigm for assessing daylight in buildings," Lighting Research & Technology, vol. 37, no. 1, pp. 41-59, 2005.
[8] L. I. Trevisan, Design, construction, and applicability verification of a Cost-effective Bioclimatic Building Chamber in building environment comfort studies, Curitiba: Federal Univerisity of Technology - Paraná, 2019.
[9] I. O. f. Standardization, ISO 7726 - Thermal environments - Instruments and methods for measuring physical quantities, Genebra: ISO, 1985.
[10] G. d. Bem, M. Mülhbauer, P. La Roche, A. Matias, R. Almeida and E. L. Krüger, "Implementation of a dynamic thermal and illuminance control system in responsive façades: shading study," Advances in Environmental and Engineering Research, vol. 3, no. 3, 2022.